12.3 Proteins Carry Out Most Membrane Processes
✓ 3 Describe the roles of proteins in making membranes selectively permeable.
We now turn to membrane proteins, which are responsible for most of the dynamic processes carried out by membranes. Membrane lipids form a permeability barrier and thereby establish compartments, whereas specific proteins mediate nearly all other membrane functions. In particular, proteins transport chemicals and information across a membrane. Membranes that differ in function differ in their protein content. Myelin, a membrane that serves as an electrical insulator around certain nerve fibers, has a low content of protein (18%). Membranes composed almost entirely of lipids are suitable for insulation because the hydrophobic components do not conduct currents well. In contrast, the plasma membranes, or exterior membranes, of most other cells must conduct the traffic of molecules into and out of the cells and so contain many pumps, channels, receptors, and enzymes. The protein content of these plasma membranes is typically 50%. Energy-transduction membranes, such as the internal membranes of mitochondria and chloroplasts, have the highest content of protein—typically, 75%. In general, membranes performing different functions contain different kinds and amounts of proteins.
Proteins Associate with the Lipid Bilayer in a Variety of Ways
Membrane proteins can be classified as being either peripheral or integral on the basis of their interaction with the hydrophobic interior of the membrane (Figure 12.8). Integral membrane proteins are embedded in the hydrocarbon chains of membrane lipids, and they can be released only when the membrane is physically disrupted. In fact, most integral membrane proteins span the lipid bilayer. In contrast, peripheral membrane proteins are bound to the head groups of lipids or the exposed portions of integral membrane proteins by electrostatic and hydrogen-bond interactions. These interactions may occur on either the cytoplasmic or the extracellular side of the membrane. Other proteins are anchored to the lipid bilayer by a covalently attached hydrophobic chain, such as a fatty acid.
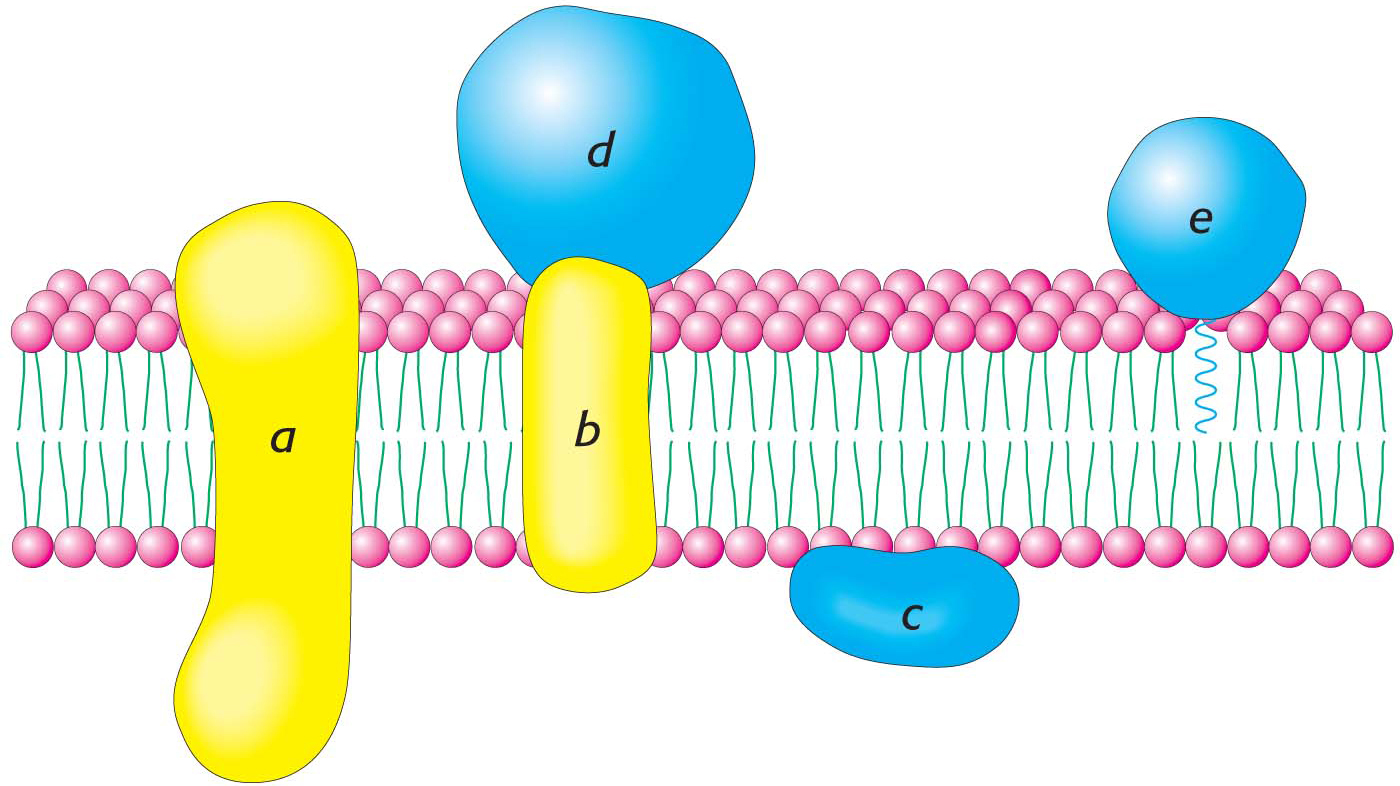
Figure 12.8: Integral and peripheral membrane proteins. Integral membrane proteins (a and b) interact extensively with the hydrocarbon region of the bilayer. Most known integral membrane proteins traverse the lipid bilayer. Some peripheral membrane proteins (c) interact with the polar head groups of the lipids. Other peripheral membrane proteins (d) bind to the surfaces of integral proteins. Some proteins (e) are tightly anchored to the membrane by a covalently attached lipid molecule.
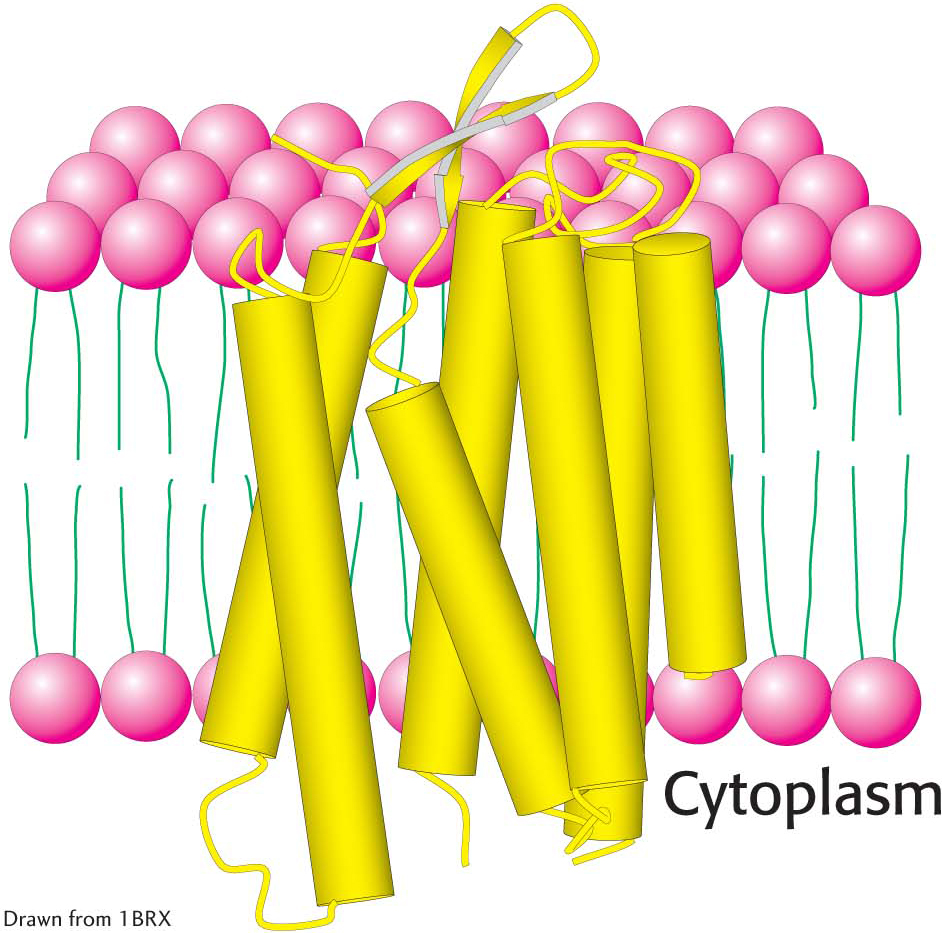
Figure 12.9: 
Structure of bacteriorhodopsin. Notice that bacteriorhodopsin consists largely of membrane-spanning α helices (represented by yellow cylinders).
Proteins can span the membrane with α helices. For instance, consider bacteriorhodopsin, which uses light energy to transport protons from inside the bacterial cell to outside, generating a proton gradient used to form ATP (Figure 12.9). Bacteriorhodopsin is built almost entirely of α helices; seven closely packed α helices, arranged almost perpendicularly to the plane of the cell membrane, span its width. Just as the nonpolar hydrocarbon moieties of phospholipids associate with one another, the nonpolar α helices of bacteriorhodopsin associate with the hydrocarbon core of the lipid bilayer. Membrane-spanning α helices are the most common structural motif in integral membrane proteins.
The membrane-spanning parts of proteins can also be formed from β strands. Porin, a protein from the outer membranes of bacteria such as E. coli, represents a class of membrane proteins that are built from β strands and contain essentially no α helices (Figure 12.10). The arrangement of β strands is quite simple: each strand is hydrogen-bonded to its neighbor in an antiparallel arrangement, forming a single β sheet. The β sheet curls up to form a hollow cylinder that, as the protein’s name suggests, forms a pore, or channel, in the membrane connecting the environment with the periplasmic space. The outside surface of porin is appropriately nonpolar, given that it interacts with the hydrocarbon core of the membrane. In contrast, the inside of the channel is quite hydrophilic and is filled with water.
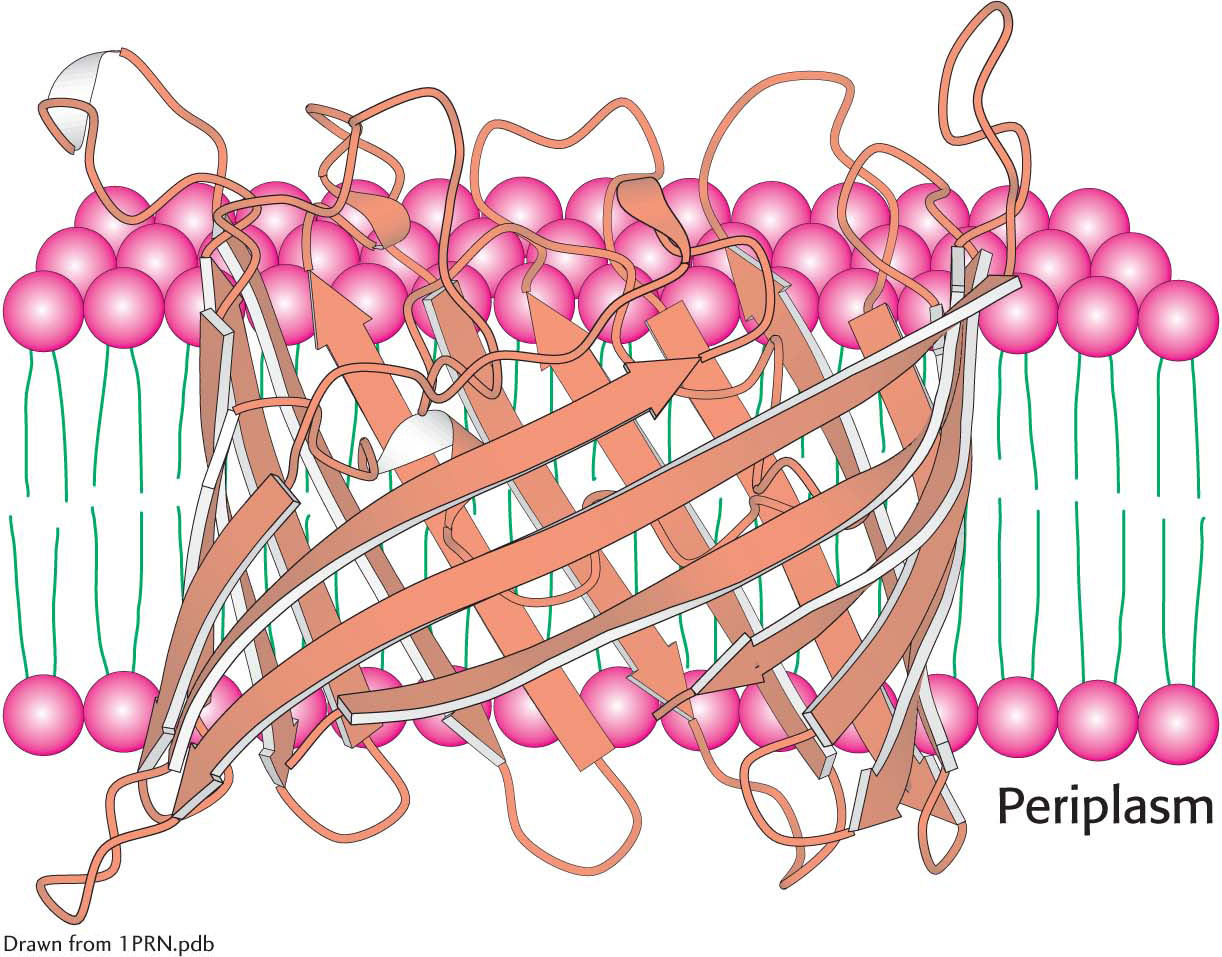
Figure 12.10: 
The structure of bacterial porin (from Rhodopseudomonas blastica). Notice that this membrane protein is built entirely of β strands. Only one monomer of the trimeric protein is shown.
Another means by which a protein associates with a membrane is the embedding of just part of the protein into the membrane. The structure of the endoplasmic reticulum membrane-bound enzyme prostaglandin H2 synthase-1 exemplifies this type of association. Prostaglandin H2 synthase-1 lies along the outer surface of the membrane but is firmly bound by a set of α helices with hydrophobic surfaces that extend from the bottom of the protein into the membrane (Figure 12.11).
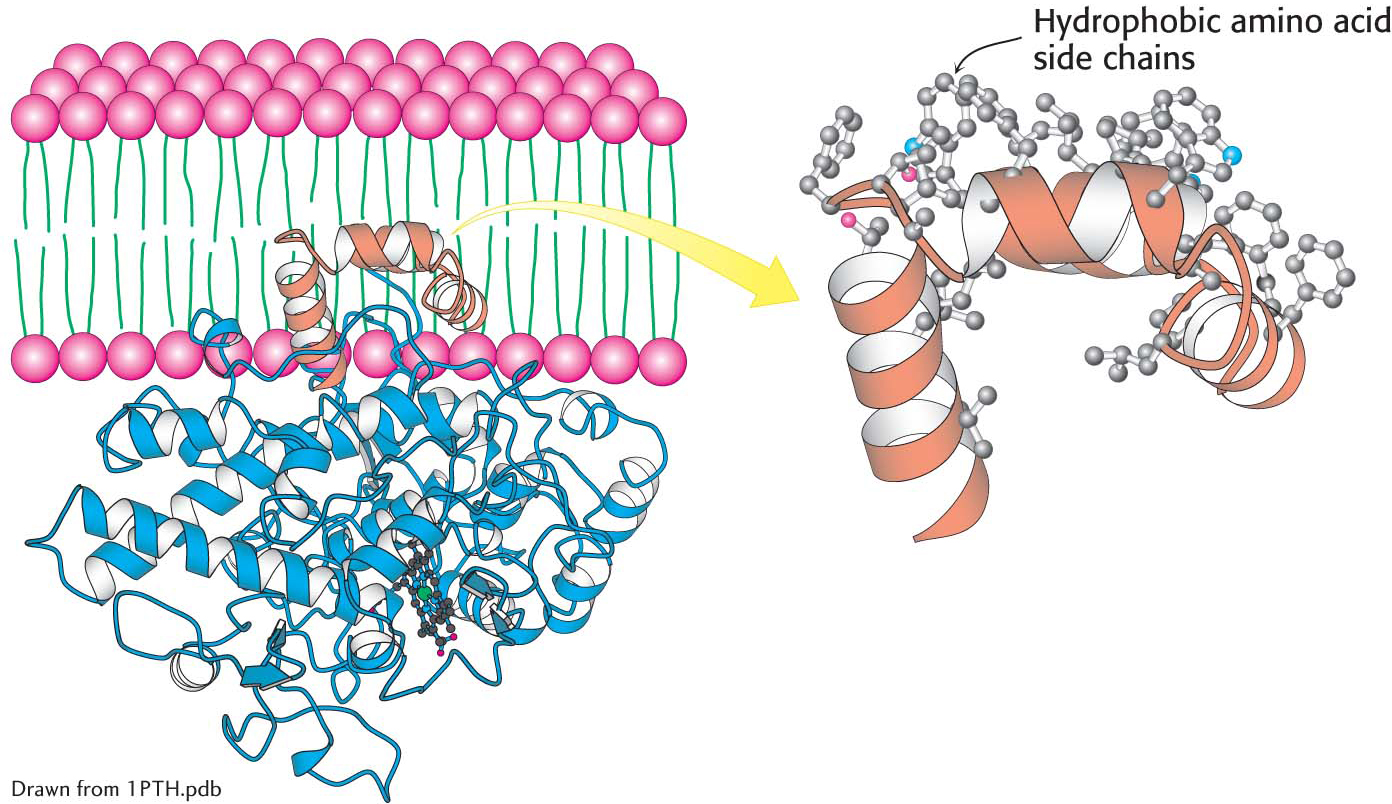
Figure 12.11:
The attachment of prostaglandin H2 synthase-1 to the membrane. Notice that prostaglandin H2 synthase-1 is held in the membrane by a set of α helices (orange) coated with hydrophobic side chains. One monomer of the dimeric enzyme is shown.
!clinic! CLINICAL INSIGHT: The Association of Prostaglandin H2 Synthase-l with the Membrane Accounts for the Action of Aspirin
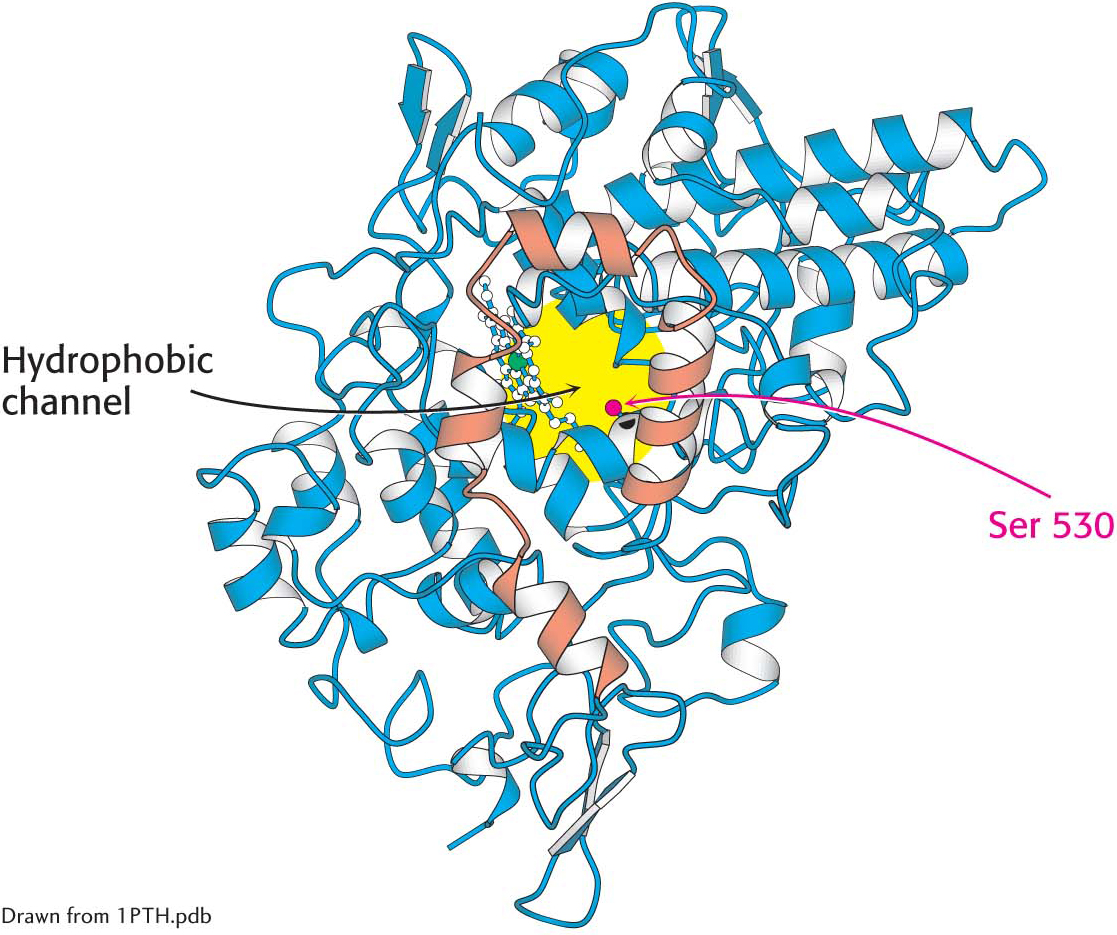
Figure 12.12:
The hydrophobic channel of prostaglandin H2 synthase-1. A view of prostaglandin H2 synthase-1 from the membrane reveals the hydrophobic channel that leads to the active site. The membrane-anchoring helices are shown in orange.
The localization of prostaglandin H2 synthase-l in the membrane is crucial to its function. Prostaglandin H2 synthase-1 catalyzes the conversion of arachidonic acid into prostaglandin H2, which promotes inflammation and modulates gastric acid secretion, in a two-step process requiring a cyclooxygenase (COX) activity and a peroxidase activity. Arachidonic acid is a fatty acid generated by the hydrolysis of membrane lipids. Arachidonic acid moves from the nonpolar core of the lipid bilayer, where it is generated, to the active site of the enzyme, without entering an aqueous environment, by traveling through a hydrophobic channel in the protein (Figure 12.12). Indeed, nearly all of us have experienced the importance of this channel leading to the cyclooxygenase: drugs such as aspirin and ibuprofen block the channel and prevent prostaglandin synthesis, thereby reducing the inflammatory response. Aspirin donates an acetyl group to a serine residue (Ser 530) that lies along the path to the active site, thereby blocking the channel (Figure 12.13). Thus, aspirin and similar drugs are called COX inhibitors.
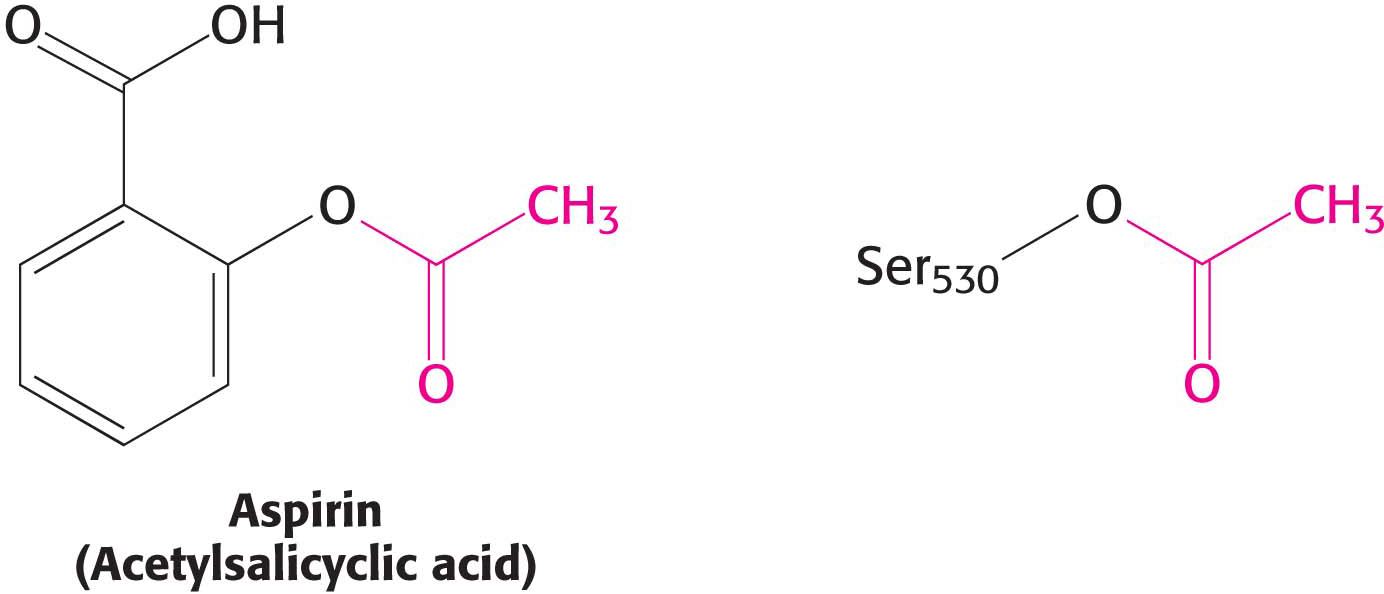
Figure 12.13: Aspirin’s effects on prostaglandin H2 synthase-1. Aspirin acts by transferring an acetyl group to a serine residue in prostaglandin H2 synthase-1.