1.1 Living Systems Require a Limited Variety of Atoms and Molecules
Ninety naturally occurring elements have been identified, yet only three—oxygen, hydrogen, and carbon—make up 98% of the atoms in an organism. Moreover, the abundance of these three elements in life is vastly different from their abundance in Earth’s crust (Table 1.1). What can account for the disparity between what is available and what organisms are made of?
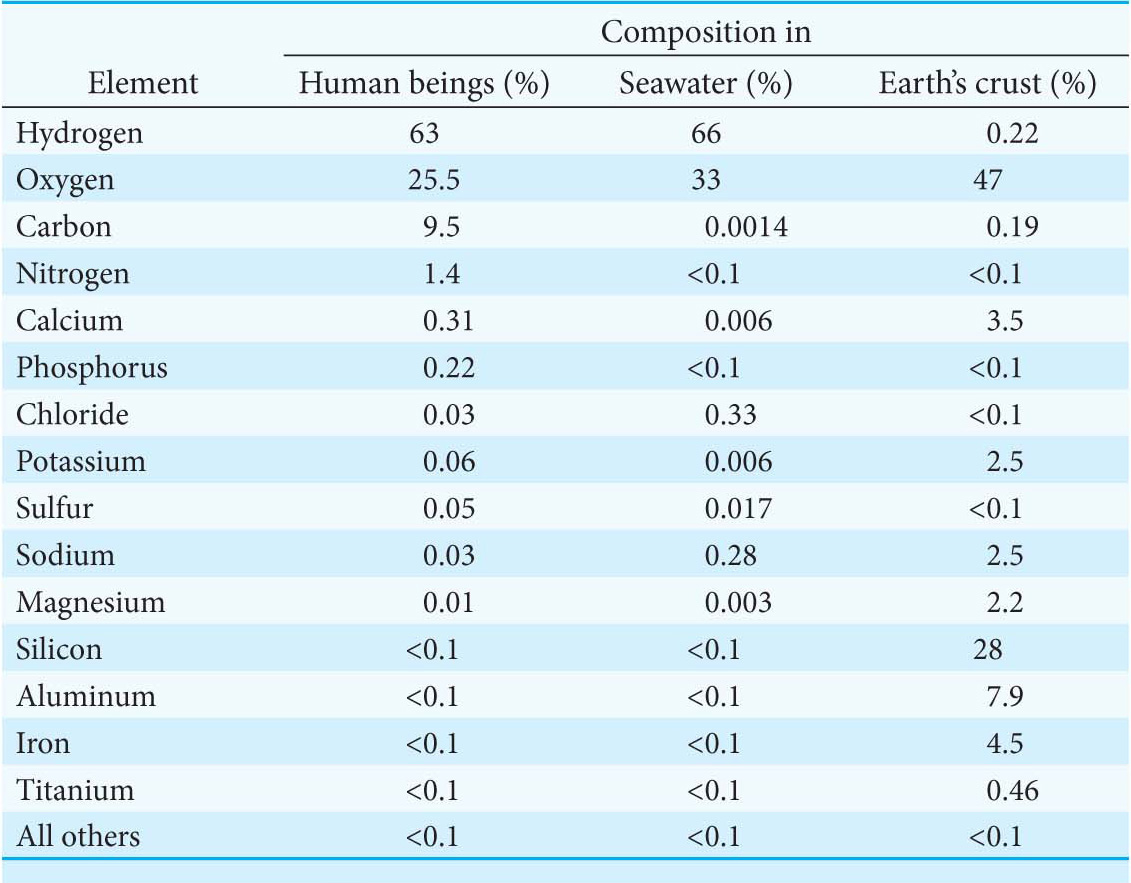
Table 1.1 Chemical compositions as percentage of total number of atoms
Note: Because of rounding, total percentages do not equal 100%. Source: Data from E. Frieden, The chemical elements of life, Sci. Am. 227(1), 1972, p. 54.
One reason that oxygen and hydrogen are so common is the ubiquity of water, or “the matrix of life,” as biochemist Albert Szent-Györgi called it. This tiny molecule—consisting of only three atoms—makes life on Earth possible. Indeed, current belief is that all life requires water, which is why so much effort has been made in recent decades to determine whether Mars had water in the past and whether it still does. The importance of water for life is so crucial that its presence is tantamount to saying that life could be present. We will consider the properties of water and how these properties facilitate biochemistry in Chapter 2.
After oxygen and hydrogen, the next most-common element in living organisms is carbon. Most large molecules in living systems are made up predominantly of carbon. Fuel molecules are made entirely of carbon, hydrogen, and oxygen. Biological fuels, like the fuels that power machinery, react with oxygen to produce carbon dioxide and water. In regard to biological fuels, this reaction, called combustion, provides the energy to power the cell. As a means of seeing why carbon is uniquely suited for life, let us compare it with silicon, its nearest elemental relative. Silicon is much more plentiful than carbon in Earth’s crust (Table 1.1), and, like carbon, can form four covalent bonds—a property crucial to the construction of large molecules. However, carbon-to-carbon bonds are stronger than silicon-to-silicon bonds. This difference in bond strength has two important consequences. First, large molecules can be built with the use of carbon–carbon bonds as the backbone because of the stability of these bonds. Second, more energy is released when carbon–carbon bonds undergo combustion than when silicon reacts with oxygen. Thus, carbon-based molecules are stronger construction materials and are better fuels than silicon-based molecules. Carbon even has an advantage over silicon after it has undergone combustion. Carbon dioxide is readily soluble in water and can exist as a gas; thus, it remains in biochemical circulation, given off by one tissue or organism to be used by another tissue or organism. In contrast, silicon is essentially insoluble after reaction with oxygen. After it has combined with oxygen, it is permanently out of circulation. Quartz is a common form of silicon dioxide.
Other elements have essential roles in living systems—notably, nitrogen, phosphorus, and sulfur. Moreover, some of the trace elements, although present in tiny amounts compared with oxygen, hydrogen, and carbon, are absolutely vital to a number of life processes. We will see specific uses of these elements as we proceed with our study of biochemistry.