22.3 Two Photosystems Generate a Proton Gradient and NADPH
✓ 2 Identify the key products of the light reactions.
✓ 3 Explain how redox balance is maintained during the light reactions.
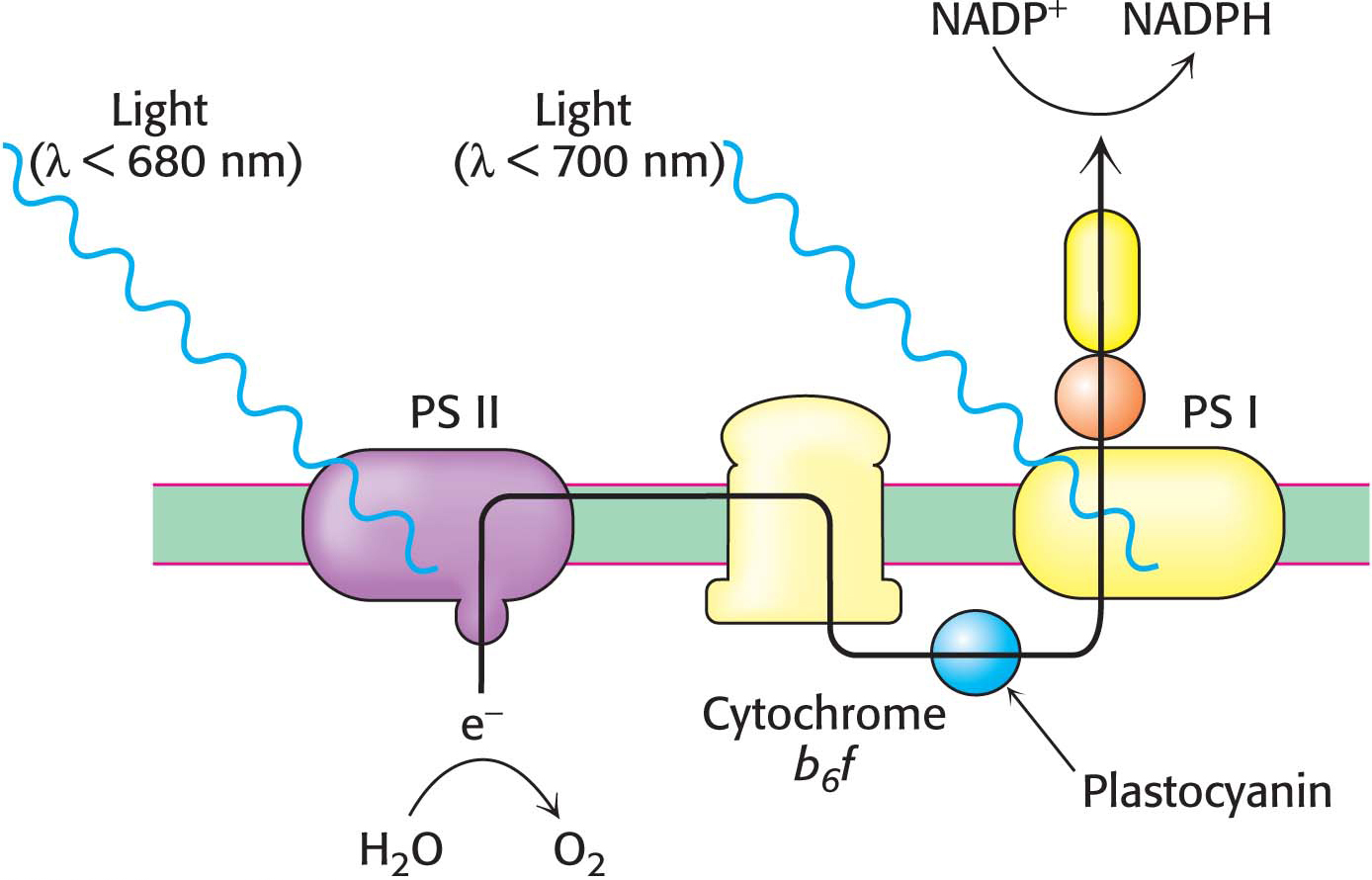
Figure 22.10: Two photosystems. The absorption of photons by two distinct photosystems (PS I and PS II) is required for complete electron flow from water to NADP+.
With an understanding of the principles of how photosynthetic organisms generate high-energy electrons, let us examine the biochemical systems that coordinate the electron capture and their use to generate reducing power and ATP, resources that will be used to power the synthesis of glucose from CO2. Photosynthesis in green plants is mediated by two kinds of membrane-bound, light-sensitive complexes—photosystem I (PS I) and photosystem II (PS II), each with its own characteristic reaction center (Figure 22.10). Photosystem I responds to light with wavelengths shorter than 700 nm and is responsible for providing electrons to reduce NADP+ to NADPH, a versatile reagent for driving biosynthetic processes requiring reducing power. Photosystem II responds to wavelengths shorter than 680 nm, sending electrons through a membrane-bound proton pump called cytochrome b6 f and then on to photosystem I to replace the electrons donated by PS I to NADP+. The electrons in the reaction center of photosystem II are replaced when two molecules of H2O are oxidized to generate a molecule of O2. As we will soon see, electrons flow from water through photosystem II, the cytochrome b6 f complex, and photosystem I and are finally accepted by NADP+. In the course of this flow, a proton gradient is established across the thylakoid membrane. This proton gradient is the driving force for ATP production.
Photosystem I Uses Light Energy to Generate Reduced Ferredoxin, a Powerful Reductant
The first stage of the light reactions is catalyzed by photosystem I (Figure 22.11). Photosystem I typically includes 14 polypeptide chains and multiple associated proteins and cofactors. The core of this system is a pair of similar subunits, PsaA (83 kDa) and PsaB (82 kDa), which bind 80 chlorophyll molecules as well as other redox factors. A special pair of chlorophyll a molecules lies at the center of the structure and absorbs wavelengths of light that are longer than those absorbed by chlorophyll a not associated with a photosystem. The special pair of the reaction center of photosystem I are called P700. When activated by light (designated P700*), the reaction center initiates photoinduced charge separation that generates the high-energy electrons. The electrons flow down an electron-transport chain through a chlorophyll called A0 and a quinone called A1 to a set of 4Fe-4S clusters. From there, the electrons are transferred to ferredoxin (Fd), a protein containing a 2Fe-2S cluster coordinated to four cysteine residues (Figure 22.12).
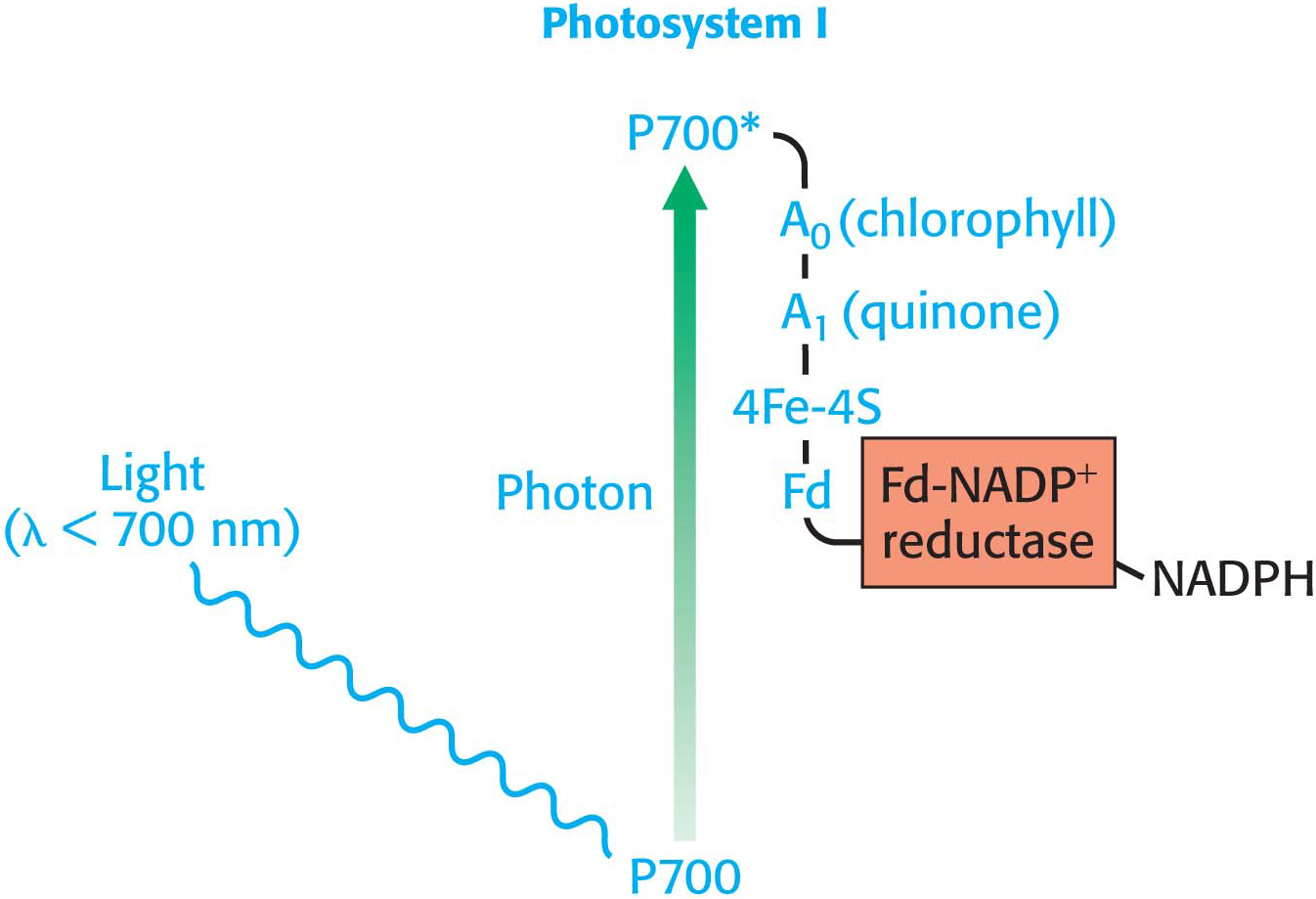
Figure 22.11: Photosystem I. Light absorption induces electron transfer from P700 down an electron-transport chain that includes a chlorophyll molecule, a quinone molecule, and three 4Fe-4S clusters to reach ferredoxin. The electron donating pigment is denoted as P700*.
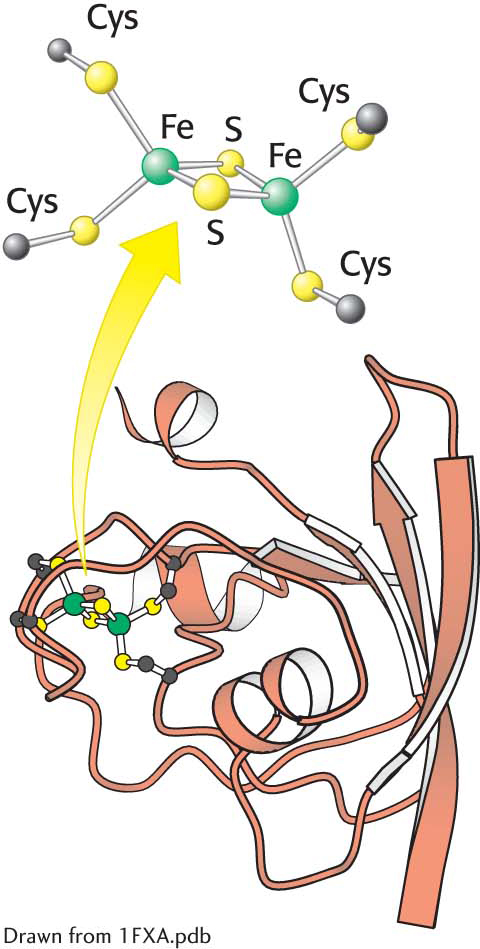
Figure 22.12:
The structure of ferredoxin. In plants, ferredoxin contains a 2Fe-2S cluster. This protein accepts electrons from photosystem I and carries them to ferredoxin-NADP+ reductase.
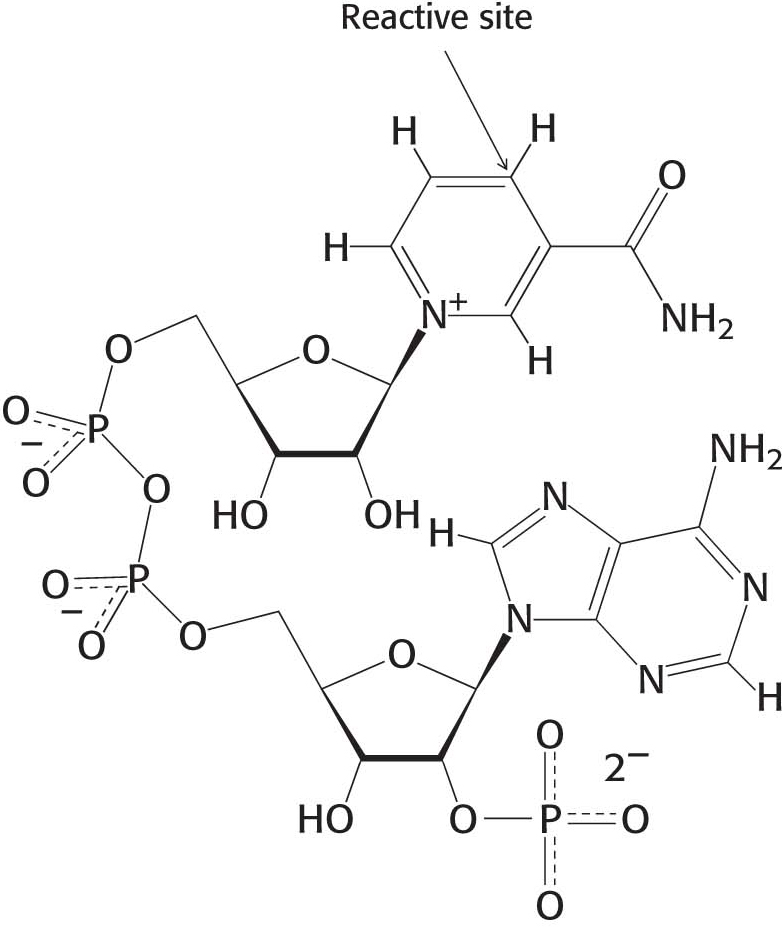
Figure 22.13: The structure of NADP+. Nicotinamide adenine dinucleotide phosphate (NADP+) is a prominent carrier of high-energy electrons. NADP+ binds a hydride ion (H−) at the reactive site to form NADPH in a reaction entirely analogous to the reduction of NAD+ (Figure 15.12).
Although reduced ferredoxin is a strong reductant, it is not useful for driving many reactions, in part because ferredoxin carries only one available electron. The currency of readily available biosynthetic reducing power in cells is NADPH, which shuttles two electrons as a hydride ion. Indeed, NADPH functions exactly as NADH does and differs structurally only in that it contains a phosphoryl group on the 2′-hydroxyl group of one of the ribose units (Figure 22.13). This phosphoryl groups acts as a label identifying NADPH as a reductant for biosynthetic (anabolic) reactions, and so it is not oxidized by the respiratory chain as is NADH. This distinction holds true in all biochemical systems, including that of human beings.
How can reduced ferredoxin be used to drive the reduction of NADP+ to NADPH? This reaction is catalyzed by ferredoxin-NADP+ reductase, a flavoprotein. The bound FAD component in this enzyme collects two electrons, one at a time, from two molecules of reduced ferredoxin as it proceeds from its oxidized form to its fully reduced form (Figure 22.14). The enzyme then transfers a hydride ion to NADP+ to form NADPH. Note that this reaction takes place on the stromal side of the thylakoid membrane. The use of a stromal proton in the reduction of NADP+ makes the stroma more basic than the thylakoid lumen and thus contributes to the formation of the proton-motive force across the thylakoid membrane that will be used to synthesize ATP. The first of the raw materials required to reduce CO2—biosynthetic reducing power as NADPH—has been generated. However, photosystem I is now deficient in electrons. How can these electrons be replenished?
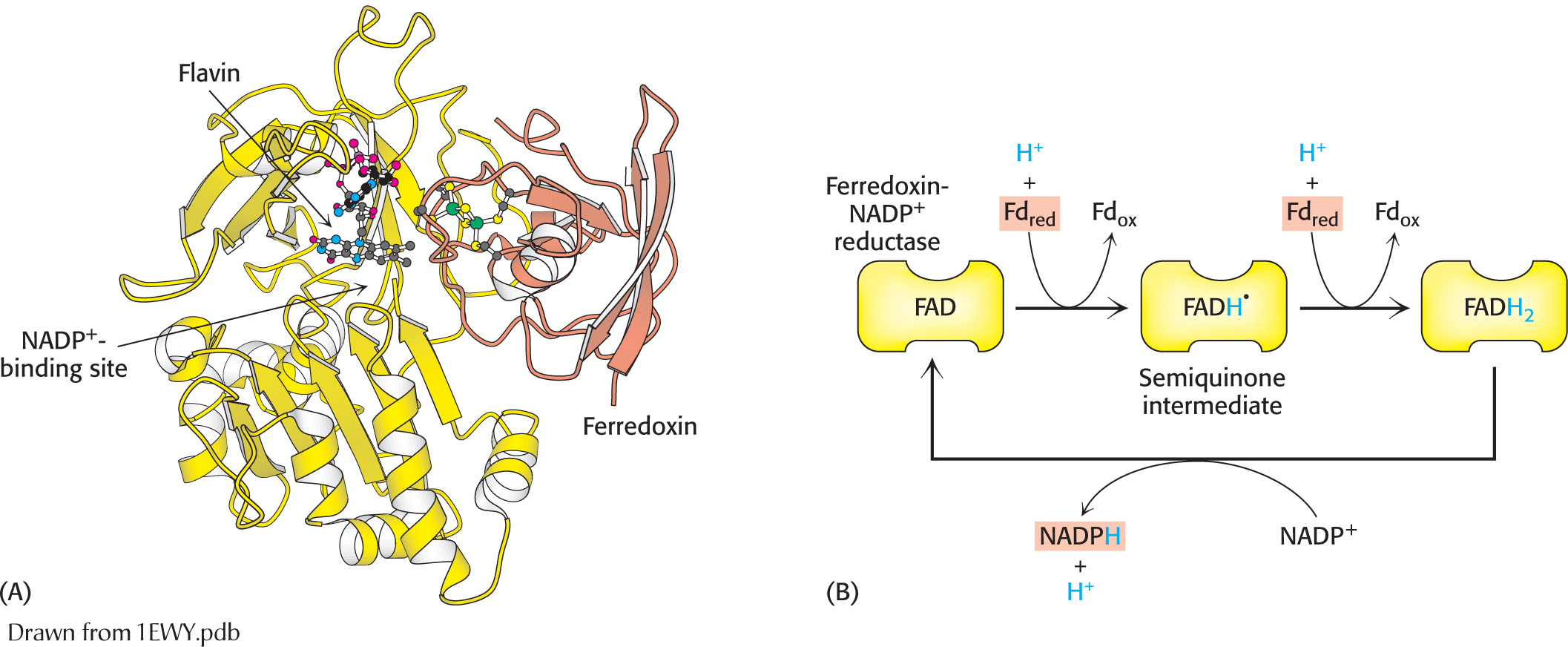
Figure 22.14:
The structure and function of ferredoxin-NADP+ reductase. (A) The structure of ferredoxin-NADP+ reductase is shown in yellow. This enzyme accepts electrons, one at a time, from ferredoxin (shown in orange). (B) Ferredoxin-NADP+ reductase first accepts two electrons and two protons from two molecules of reduced ferredoxin (Fdred) to form FADH2, which then transfers two electrons and a proton to NADP+ to form NADPH.
Photosystem II Transfers Electrons to Photosystem I and Generates a Proton Gradient
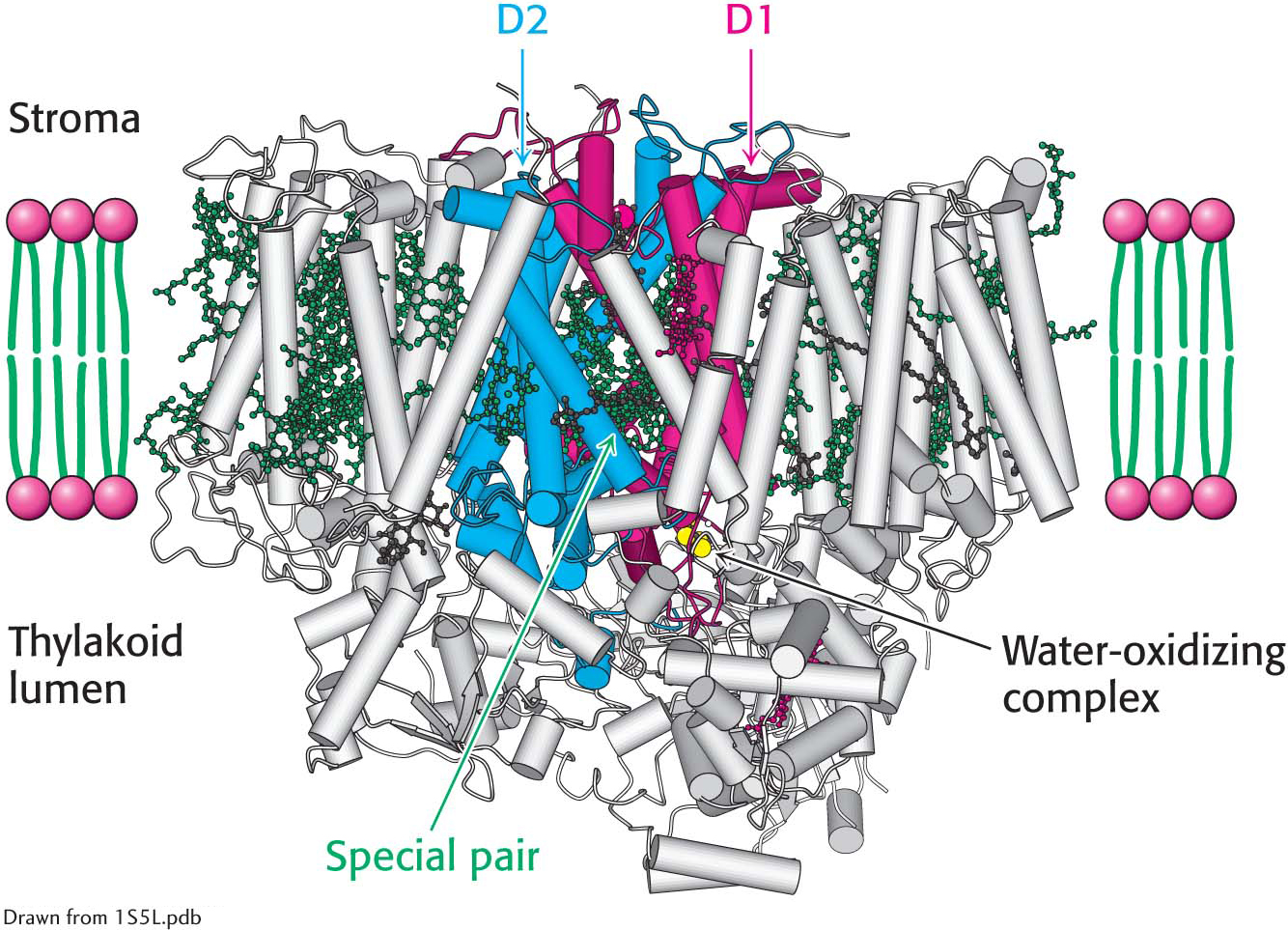
Figure 22.15:
The structure of photosystem II. The D1 and D2 subunits are shown in red and blue, respectively, and the numerous bound chlorophyll molecules are shown in dark green. Notice that the special pair and the water-oxidizing complex (the site of oxygen evolution) lie toward the thylakoid-lumen side of the membrane.
DID YOU KNOW?
Pheophytin is formed in the cooking of some green vegetables when the magnesium ion of chlorophyll is lost and replaced by protons. The absorption properties of pheophytin are different from those of the chlorophylls, often resulting in cooked vegetables that are a dull green compared with their uncooked counterparts.
Restocking the electrons of photosystem I requires another source of electrons. One of the roles of photosystem II is to provide electrons to photosystem I. Photosystem II catalyzes the light-driven transfer of electrons from water to photosystem I. In the process, protons are pumped into the thylakoid lumen to generate a proton-motive force. Photosystem II is an enormous assembly of more than 20 subunits. It is formed by D1 and D2, a pair of similar 32-kDa proteins that span the thylakoid membrane (Figure 22.15).
The photochemistry of photosystem II begins with the excitation of a special pair of chlorophyll molecules that are often called P680, generating P680* (Figure 22.16). P680* rapidly transfers electrons to a nearby pheophytin (designated Ph), a chlorophyll with two H+ ions in place of the central Mg2+ ion. From there, the electrons are transferred first to a tightly bound plastoquinone at site QA and then to a mobile plastoquinone at site QB. Plastoquinone is an electron carrier that closely resembles ubiquinone, a component of the mitochondrial electron-transport chain. With the arrival of a second electron and the uptake of two protons, the mobile plastoquinone is reduced to plastoquinol, QH2.
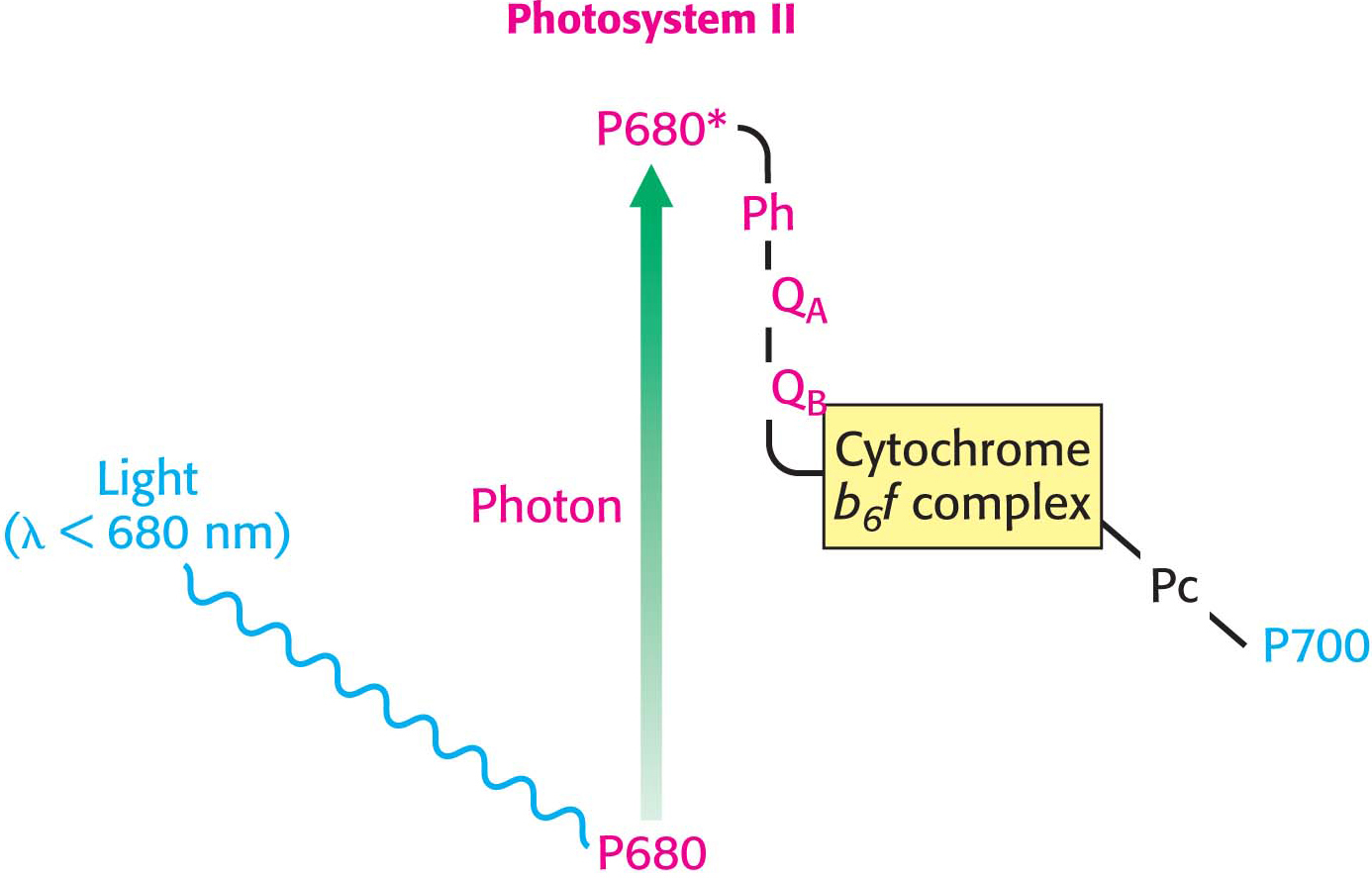
Figure 22.16: Photosystem II provides electrons to photosystem I. As electrons flow from photosystem II to photosystem I, they pass through the cytochrome b6 f complex, a proton pump. The electron donating pigment is denoted as P680*. Abbreviations: Ph, pheophytin; QA and QB, plastoquinones; Pc, plastocyanin.
The site of quinone reduction in PS II is on the side of the stroma. Thus, the two protons that are taken up with the reduction of each molecule of plastoquinone come from the stroma, increasing the proton gradient. This gradient is further augmented by the link between photosystem II and photosystem I, the cytochrome b6 f complex.
Cytochrome b6 f Links Photosystem II to Photosystem I
The QH2 produced by photosystem II transfers its electrons to plastocyanin, which in turn donates the electrons to photosystem I, thereby replenishing the missing electrons in photosystem I. The chain starts when the electrons are transferred, one at a time from QH2, to plastocyanin (Pc), a copper-containing protein in the thylakoid lumen (Figure 22.10). This reaction is catalyzed by cytochrome b6 f (plastoquinol-plastocyanin reductase), a proton pump:
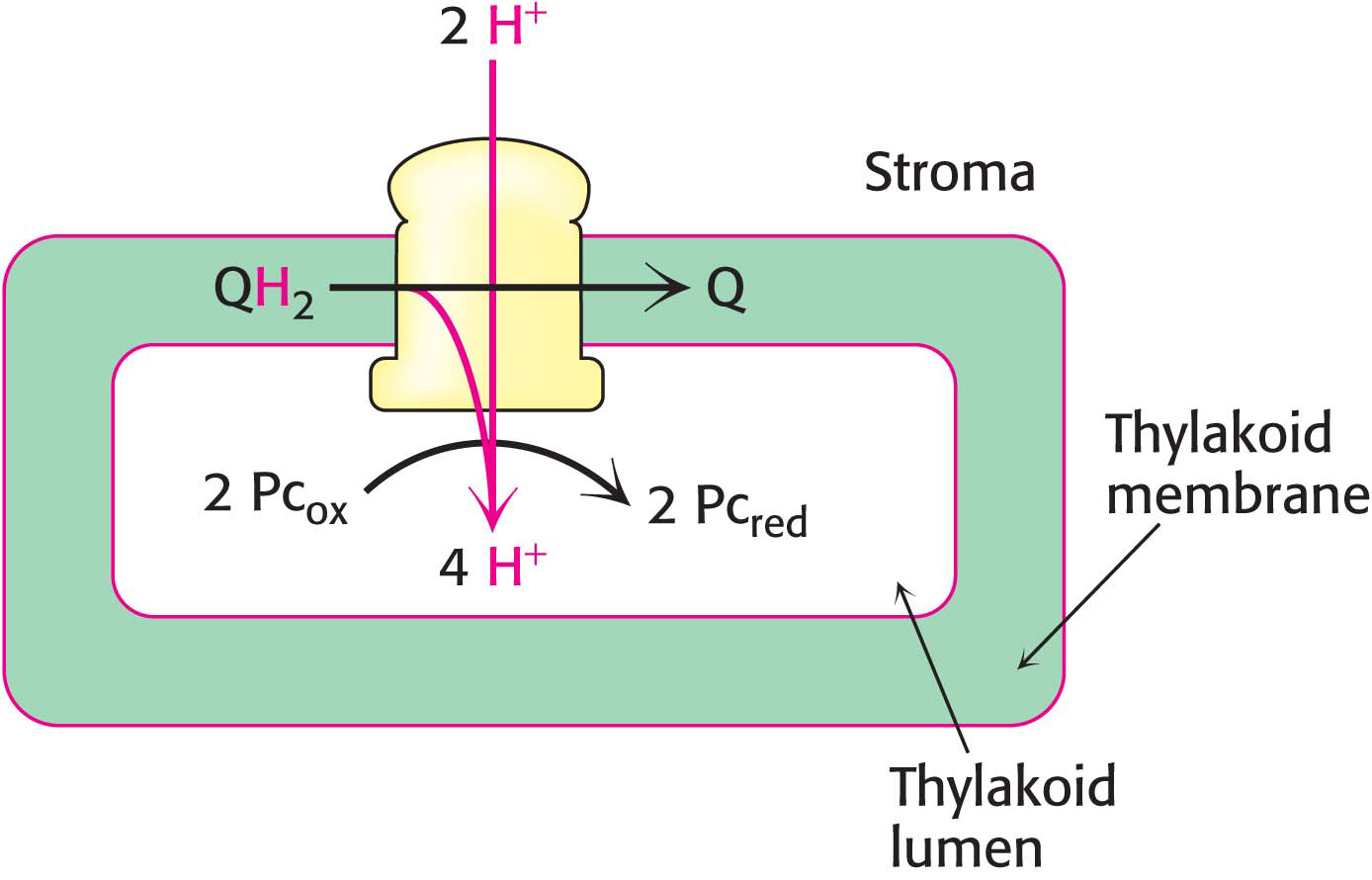
Figure 22.17: Cytochrome b6 f’s contribution to the proton gradient. The cytochrome b6 f complex (yellow structure) oxidizes QH2 to Q through the Q cycle. Four protons are released into the thylakoid lumen in each cycle.
The cytochrome b6 f reaction is reminiscent of that catalyzed by ubiquinol cytochrome c oxidoreductase in oxidative phosphorylation. Indeed, most components of the cytochrome b6 f complex are similar to those of Q-cytochrome c oxidoreductase.
The oxidation of plastoquinol by the cytochrome b6 f complex takes place by a mechanism nearly identical with the Q cycle of the electron-transport chain of cellular respiration. In photosynthesis, the net effect of the Q cycle is, first, to release protons from QH2 into the lumen and, second, to pump protons from the stroma to the lumen, strengthening the protonmotive force (Figure 22.17). Thus, electrons from photosystem II are used to replace electrons in photosystem I and, in the process, augment the proton gradient. However, the electron-deficient P680 must now be replenished with electrons.
The Oxidation of Water Achieves Oxidation–Reduction Balance and Contributes Protons to the Proton Gradient
We experience the results of attaining photosynthetic redox balance with every breath that we take. The electron-deficient P680+ is a very strong oxidant and is capable of extracting electrons from water molecules, resulting in the release of oxygen. This reaction, the photolysis of water, takes place at the water-oxidizing complex (WOC), also called the manganese center. The core of this complex includes a calcium ion, four manganese ions, and four water molecules (Figure 22.18A). Manganese is especially suitable for photolyzing water because of its ability to exist in multiple oxidation states and to form strong bonds with oxygen-containing species.
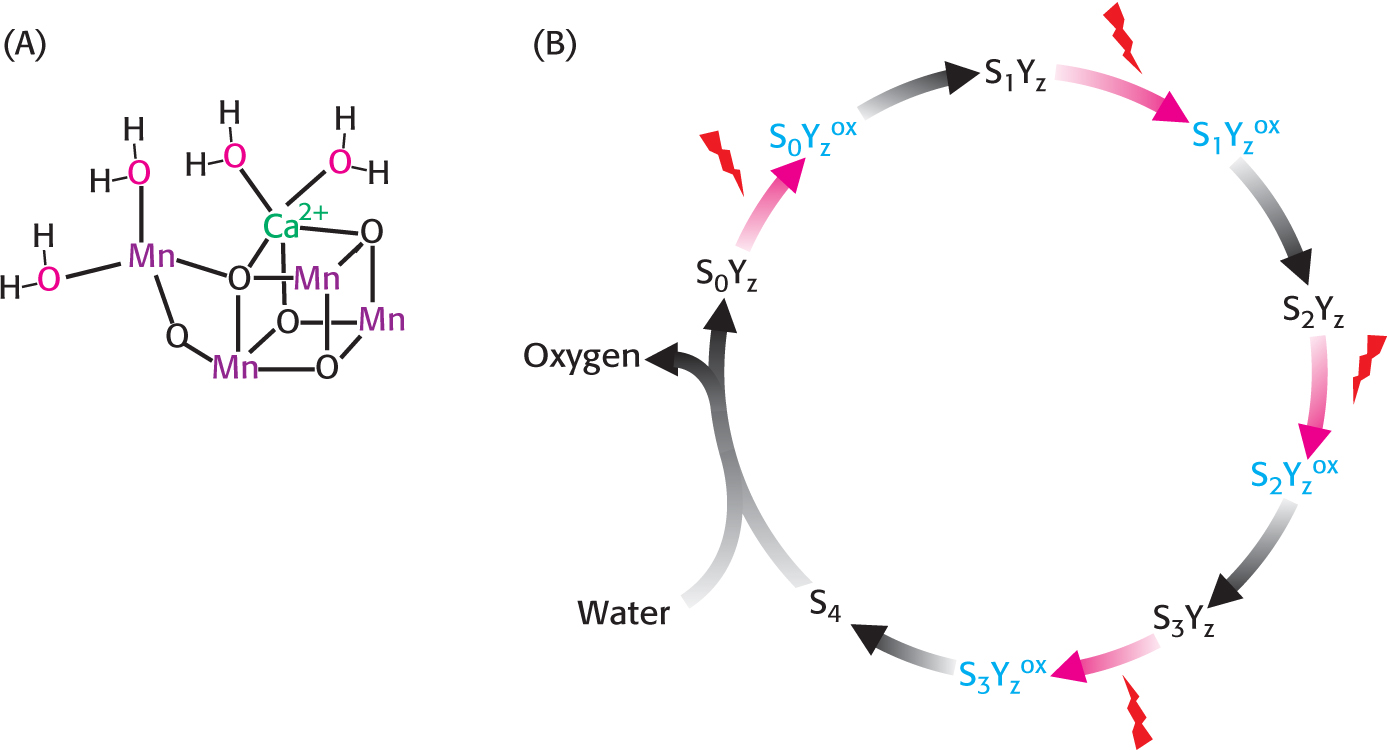
Figure 22.18: The core of the water-oxidizing complex. (A) The deduced core structure of the WOC including four manganese ions and one calcium ion is shown. The valence states of the individual manganese ions are not indicated because of uncertainty about the charge on the individual ions. The center is oxidized, one electron at a time, until two H2O molecules are linked to form a molecule of O2, which is then released from the complex. (B) The absorption of photons by the reaction center (red arrows) generates a tyrosine radical (Y), which then extracts electrons from the manganese ions. The structures are designated S0 to S4 to indicate the number of electrons that have been removed.
In its reduced form, the WOC oxidizes two molecules of water to form a single molecule of oxygen. Each time the absorbance of a photon powers the removal of an electron from P680, the positively charged special pair extracts an electron from a tyrosine residue of subunit D1 of the WOC, forming a tyrosine radical (Figure 22.18B). The tyrosine radical then removes an electron from a manganese ion. This process occurs four times, with the result that H2O is oxidized to generate O2 and H+:
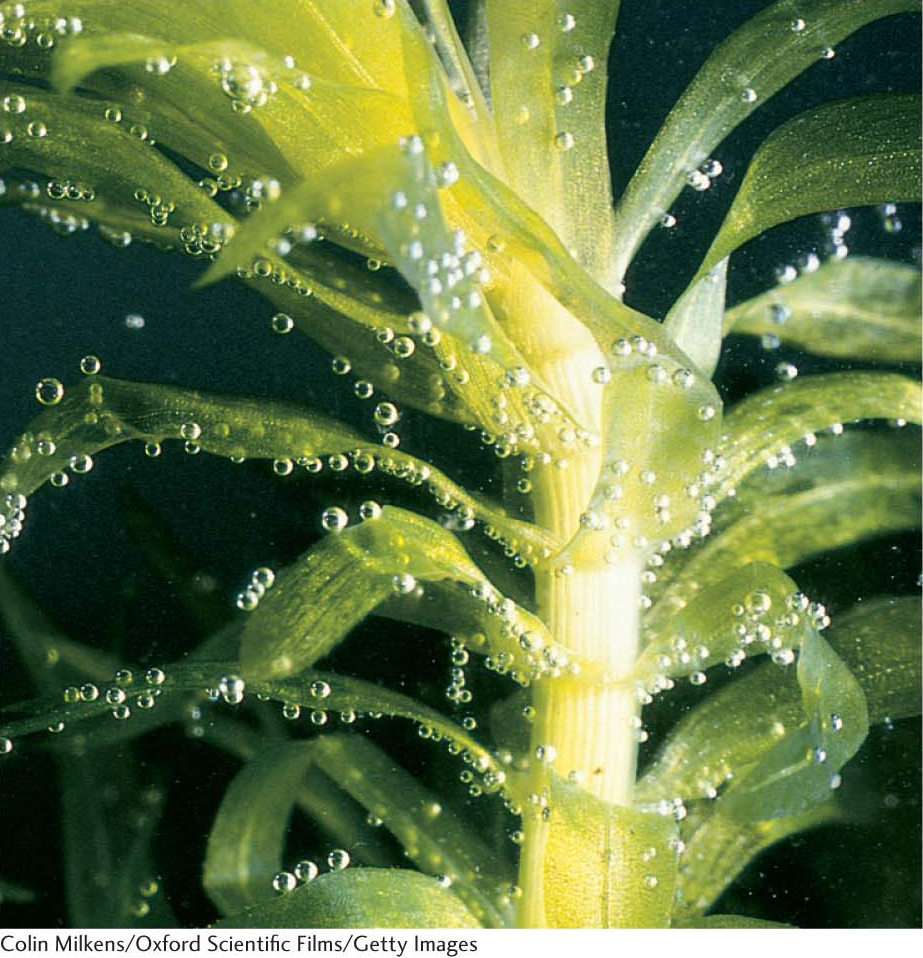
Figure 22.19: Elodea. The production of oxygen is evident by the generation of bubbles in this aquatic plant.
Four photons must be absorbed to extract four electrons from a water molecule. The four electrons harvested from water are used to reduce two molecules of Q to QH2. All oxygenic phototrophs, the most common type of photosynthetic organism, use the same inorganic core and protein components for capturing the energy of sunlight. A single solution to the biochemical problem of extracting electrons from water evolved billions of years ago, and has been conserved for use under a wide variety of phylogenetic and ecological circumstances. The photolysis of water by photosynthetic organisms is essentially the sole source of oxygen in the biosphere. This gas, so precious to our lives, is in essence a waste product of photosynthesis that results from the need to achieve redox balance (Figure 22.19).
The cooperation between photosystem I and photosystem II creates electron flow—an electrical current—from H2O to NADP+. The pathway of electron flow is called the Z scheme of photosynthesis because the redox diagram from P680 to P700* looks like the letter Z (Figure 22.20).
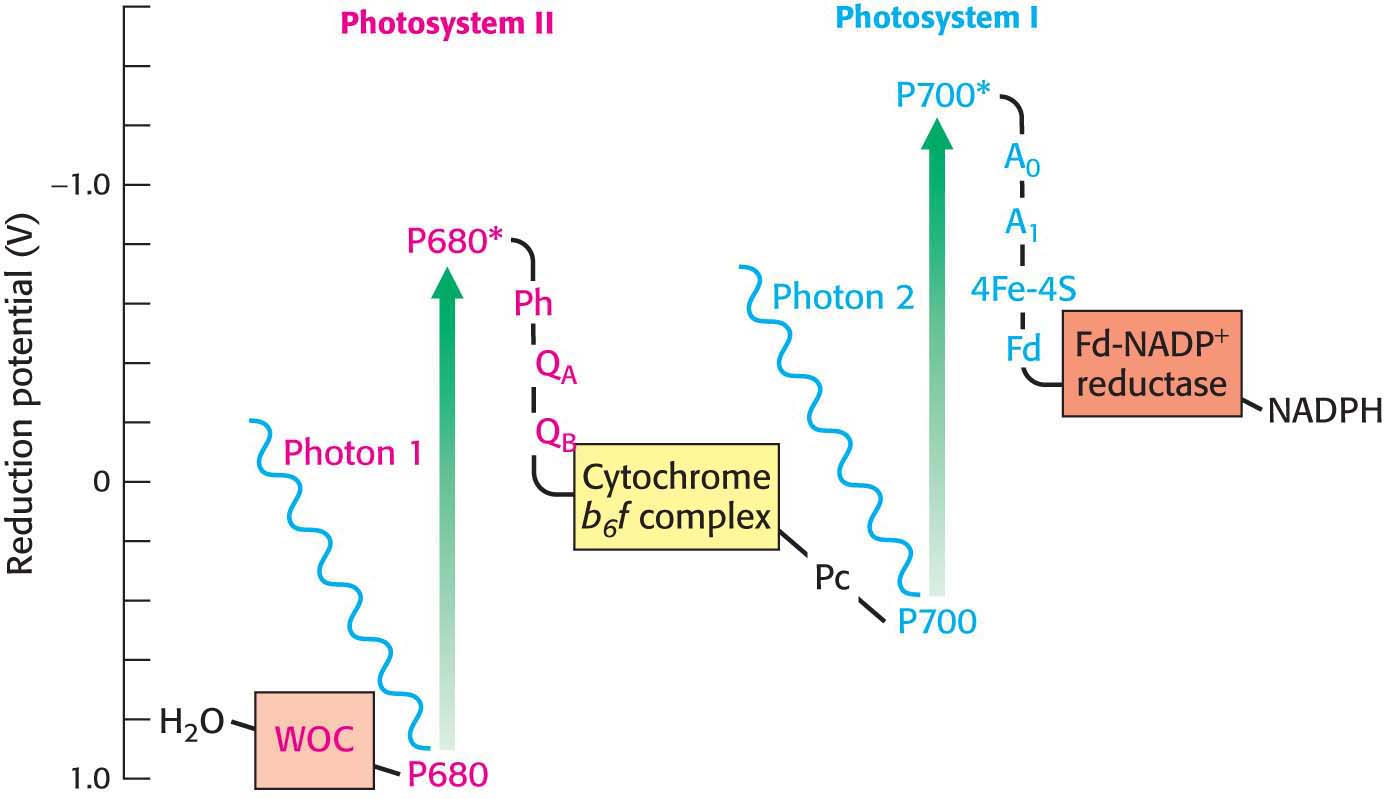
Figure 22.20: The pathway of electron flow from H2O to NADP+ in photosynthesis. This endergonic reaction is made possible by the absorption of light by photosystem II (P680) and photosystem I (P700). Abbreviations: Ph, pheophytin; QA and QB, plastoquinones; Pc, plastocyanin; A0 and A1, acceptors of electrons from P700*; Fd, ferredoxin; WOC, water-oxidizing complex.
DID YOU KNOW?
“What drives life is a little electrical current, kept up by a little sunshine.”
Nobel prize–winning biochemist
!quickquiz! QUICK QUIZ
Photosystem I produces a powerful reductant, whereas photosystem II produces a powerful oxidant. Identify the reductant and oxidant and describe their roles.
Although we have focused on the green plants, which use H2O as the electron donor in photosynthesis, Table 22.1 shows that photosynthetic bacteria display a wide variety of electron donors.
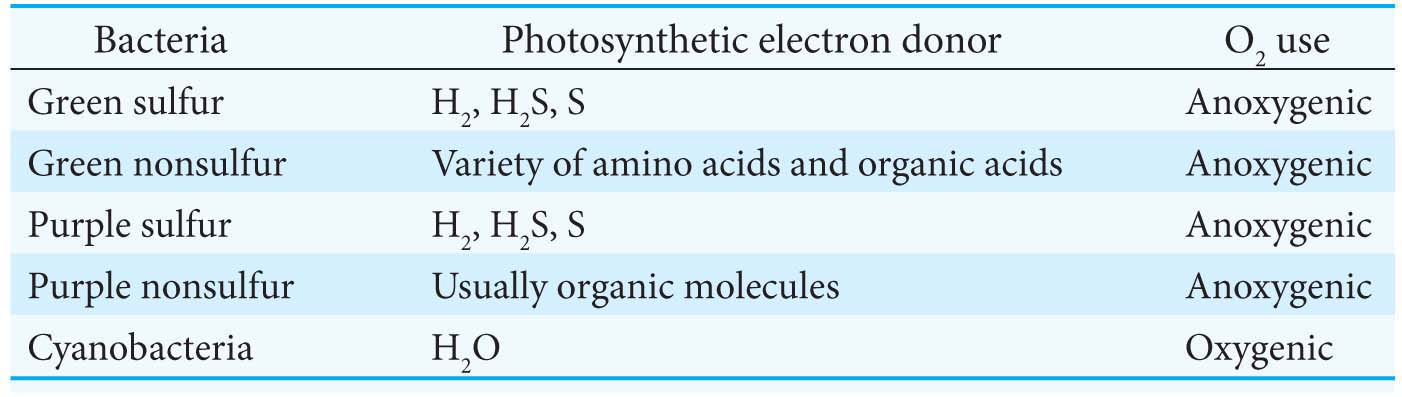
Table 22.1 Major groups of photosynthetic bacteria