33.4 Eukaryotic DNA Is Associated with Specific Proteins
✓ 2 Describe how DNA is packaged to fit inside the cell.
There are about 3.6 m of DNA in each human cell, which are packaged into 46 chromosomes, all of which are in a nucleus that has a diameter of about 5 μm. Clearly, the length of DNA and the size of the nucleus present a packaging problem. Supercoiling contributes to the compaction of DNA. However, further compaction is necessary while, at the same time, maintaining the accessibility of base-sequence information. What ways other than supercoiling exist to compact the large amount of DNA in a eukaryotic nucleus? Further compaction is attained with the assistance of certain proteins. The final DNA–protein complex is called a chromosome. We will examine one important class of proteins that takes part in the initial stages of compaction.
Nucleosomes Are Complexes of DNA and Histones
Eukaryotic DNA is tightly bound to a group of small basic proteins called histones. In fact, histones constitute half the mass of a eukaryotic chromosome. The entire complex of a cell’s DNA and associated protein is called chromatin. Chromatin is essentially isolated chromosomes, although the exact relation between the two remains to be determined. Five major histones are present in chromatin: four histones, called H2A, H2B, H3, and H4, associate with one another; the other histone is called H1. Histones have strikingly basic properties because a quarter of the residues in each histone are either arginine or lysine.
Chromatin is made up of repeating units, each containing 200 bp of DNA and two copies each of H2A, H2B, H3, and H4, called the histone octamer. These repeating units are known as nucleosomes. When chromatin is stretched out so that the DNA and associated nucleosomes can be seen by an electron microscope, it has the appearance of beads on a string; each bead has a diameter of approximately 100 Å (Figure 33.24). Partial digestion of chromatin with DNAse, an enzyme that hydrolytically cleaves the phosphodiester backbone of the DNA, yields the isolated beads. These particles consist of fragments of DNA ≈200 bp in length bound to the eight histones. More extensive digestion yields a DNA fragment of 145 bp bound to the histone octamer. This smaller complex of the histone octamer and the 145-bp DNA fragment is the nucleosome core particle. The DNA connecting core particles in undigested chromatin is called linker DNA (Figure 33.25). Histone H1 binds, in part, to the linker DNA.
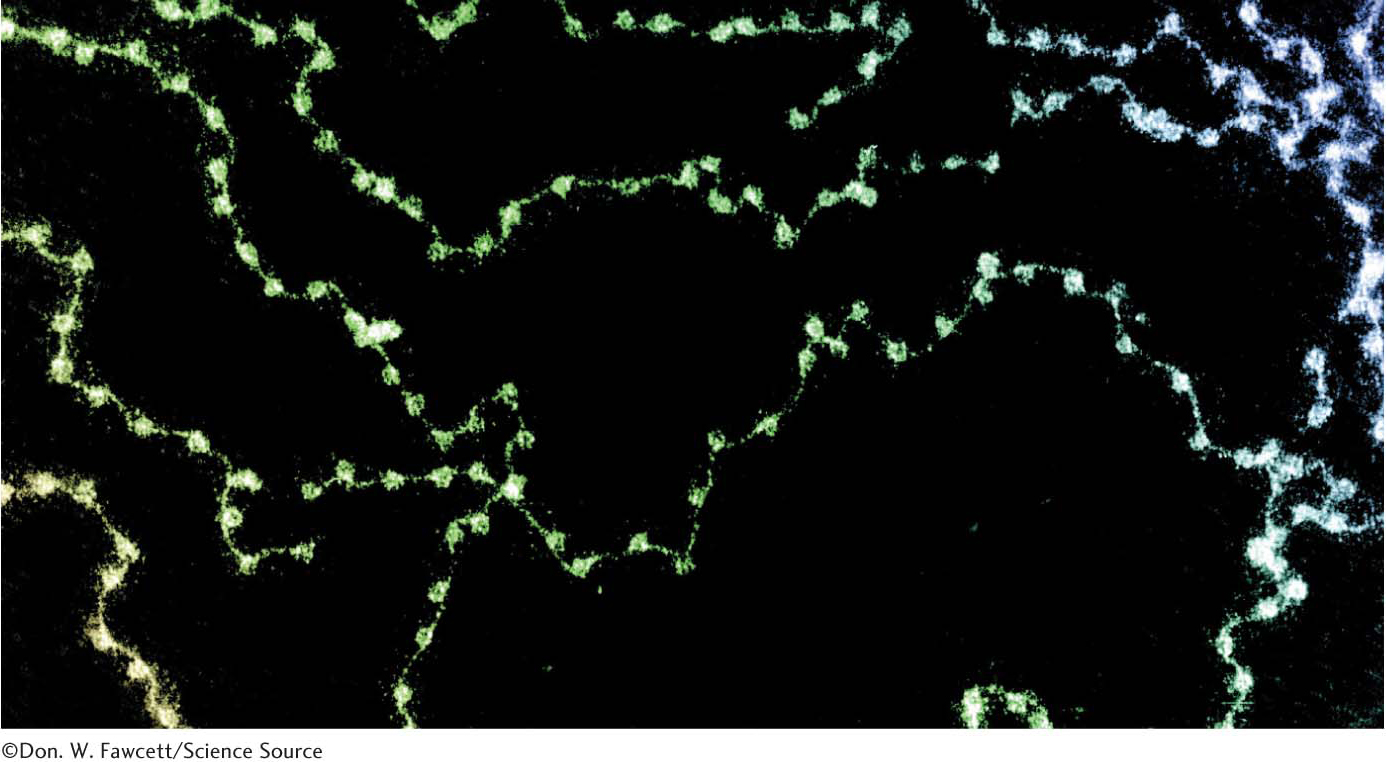
Figure 33.24: Chromatin structure. An electron micrograph of chromatin showing its “beads on a string” character.
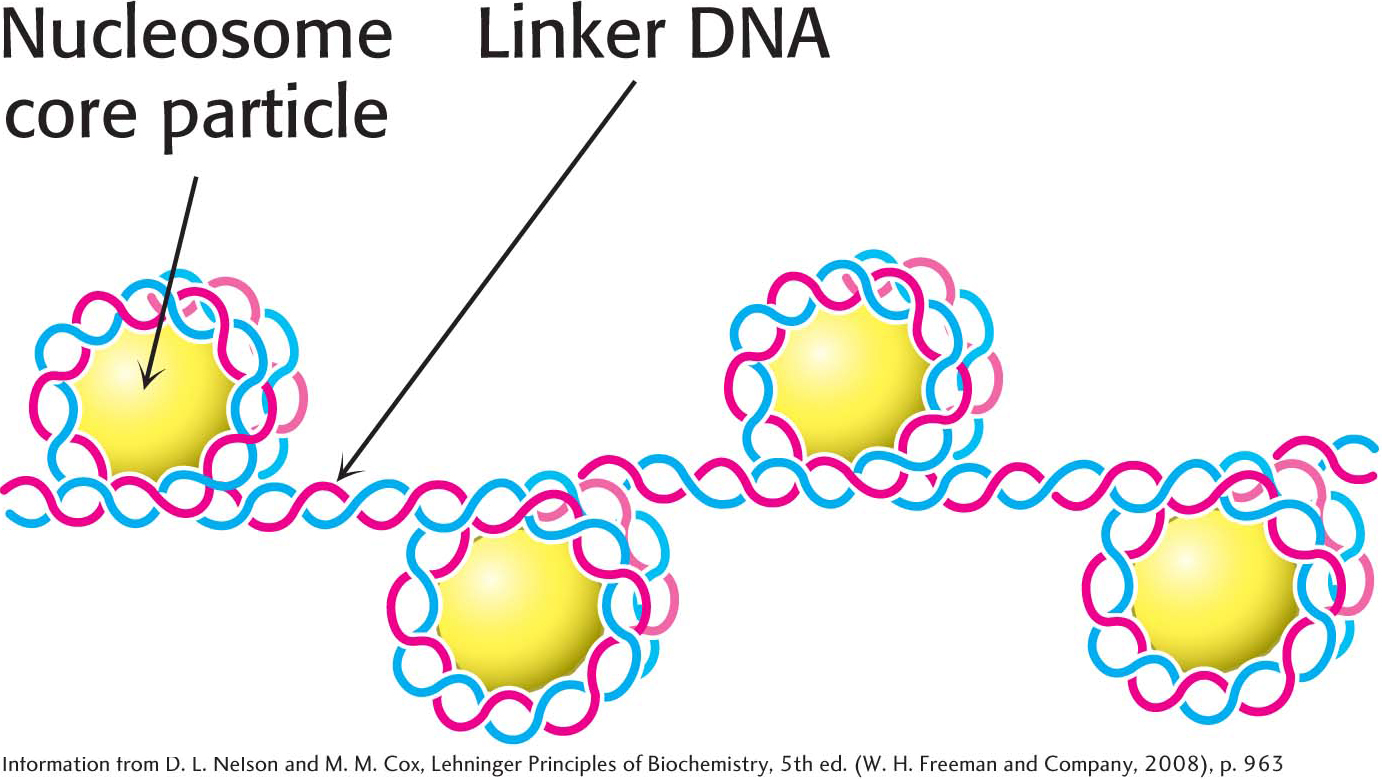
Figure 33.25: Linked core particles. Core particles are joined to one another by linker DNA.
Eukaryotic DNA Is Wrapped Around Histones to Form Nucleosomes
The eight histones in the core particle are arranged into an (H3)2(H4)2 tetramer and a pair of H2A–H2B dimers (Figure 33.26). The tetramer and dimers come together to form a complex around which the DNA wraps. In addition, each histone has an amino-terminal tail that extends out from the core structure. These tails are flexible and contain a number of lysine and arginine residues. As we shall see, covalent modifications of these tails play an essential role in modulating the accessibility of DNA for transcription (Chapter 37).
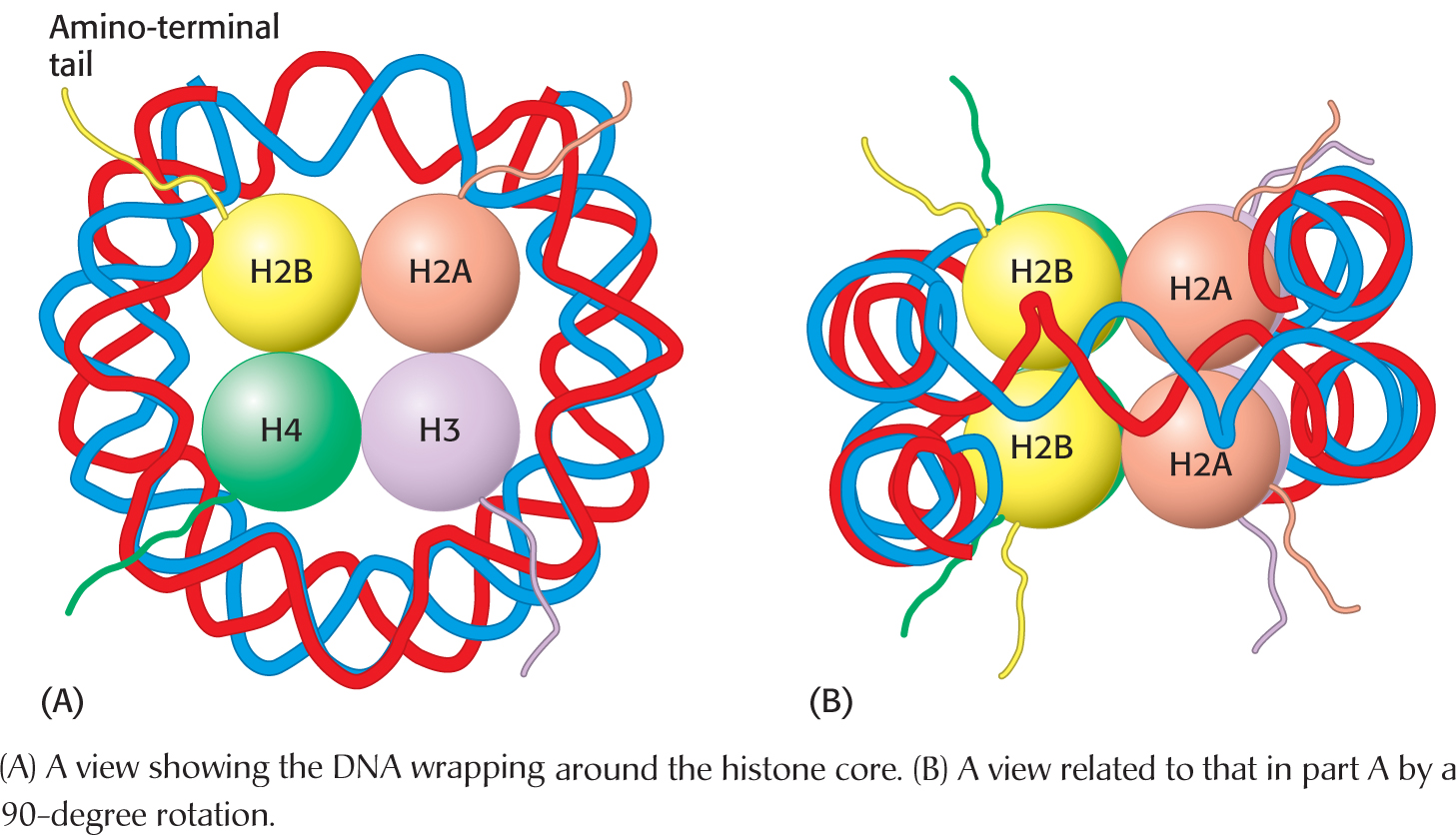
Figure 33.26: A nucleosome core particle. Schematic representations of a core of eight histone proteins surrounded by DNA.
The DNA forms a left-handed superhelix as it wraps around the outside of the histone octamer. The protein core forms contacts with the inner surface of the superhelix at many points, particularly along the phosphodiester backbone and the minor groove of the DNA. Histone H1, which has a different structure from that of the other histones, seals off the nucleosome at the location at which the linker DNA enters and leaves the nucleosome. The amino acid sequences of histones, including their amino-terminal tails, are remarkably conserved from yeast through human beings.
!quickquiz! QUICK QUIZ 2
Why are supercoiling and nucleosome formation important for the structure of DNA in a cell?
The winding of DNA around the nucleosome core contributes to DNA’s packing by decreasing its linear extent. An extended 200-bp stretch of DNA would have a length of about 680 Å. Wrapping this DNA around the histone octamer reduces the length to approximately 100 Å along the long dimension of the nucleosome. Thus, the DNA is compacted by a factor of 7. However, human chromosomes in metaphase, which are highly condensed, are compacted by a factor of 104. Clearly, the nucleosome is just the first step in DNA compaction. What is the next step? The nucleosomes themselves are arranged in 30-nm fibers. The nature of the arrangement is under investigations, and more than one arrangement is likely. In one model, the nucleosomes are packed into two interwound left-handed helical stacks. The linker DNA connects successive nucleosomes, crossing the interior of the fiber (Figure 33.27). The folding of these fibers of nucleosomes into loops further compacts DNA (Figure 33.28).
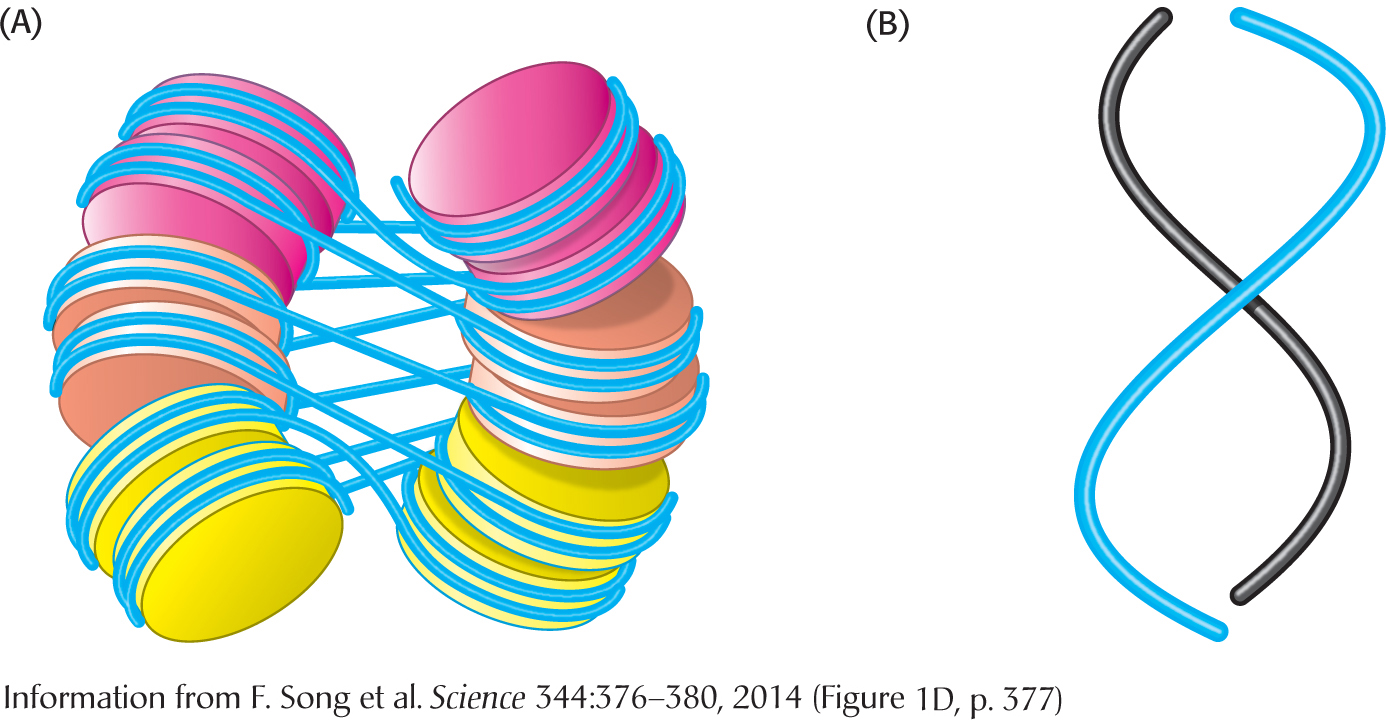
Figure 33.27: Higher-order chromatin structure. (A) A proposed model for chromatin in which the nucleosomes are packed into two interwound left-handed helical stacks. (B) The paths of two helical stacks are illustrated.
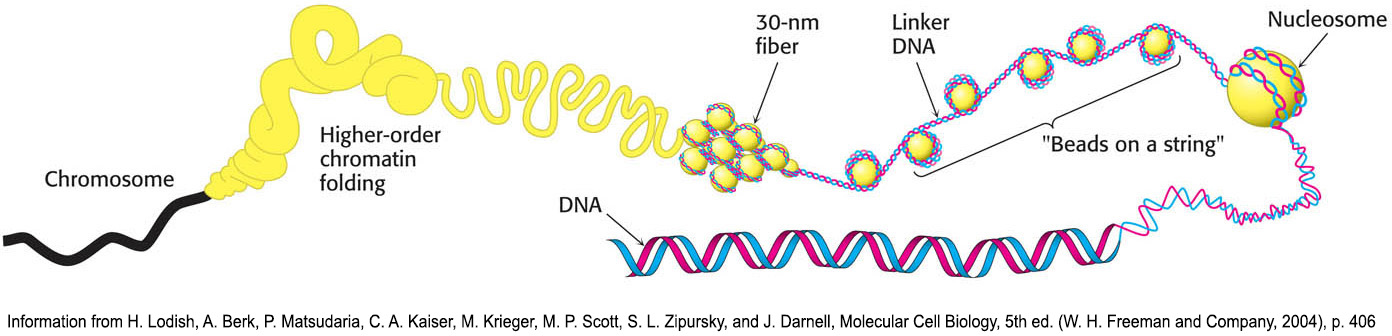
Figure 33.28: The compaction of DNA into a eukaryotic chromosome.
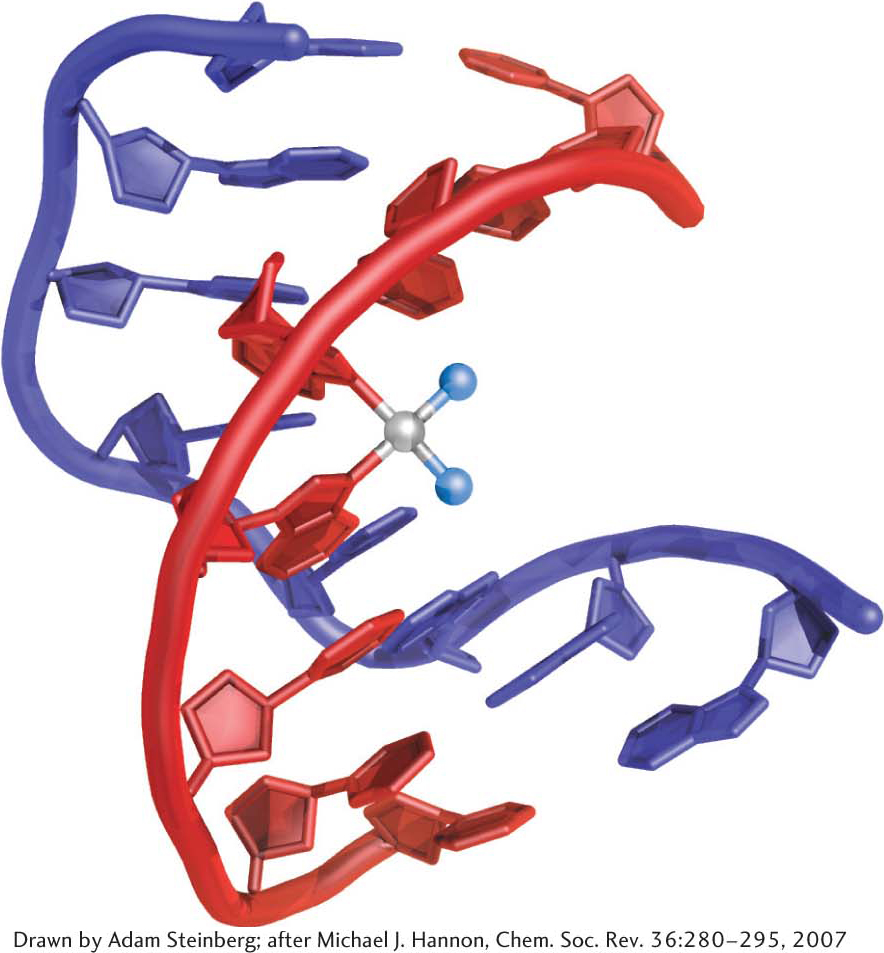
Figure 33.29: Cisplatin alters the structure of DNA. The anticancer drug cisplatin binds to DNA and disrupts its structure, leading to cell death.
!clinic! CLINICAL INSIGHT: Damaging DNA Can Inhibit Cancer-Cell Growth
Some cancer chemotherapy drugs work by modifying the structure of DNA, thereby inhibiting replication and transcription. Because cancer cells grow and divide faster than most nonmalignant cells, chemotherapy drugs have a more pronounced effect on cancer cells. An example of a chemotherapy drug is cisplatin, which is used to treat a variety of cancers, including testicular cancer and ovarian cancer. When cisplatin reacts with DNA, the chloride ligands are displaced by purine nitrogen atoms, most commonly in guanine, on two adjacent bases on the same strand. The formation of these bonds causes a severe kink in the structure of the DNA (Figure 33.29). This structural disruption prevents replication and transcription. Moreover, research results suggest that certain nuclear proteins bind to the cisplatin-damaged DNA and prevent access to DNA-repair enzymes (Chapter 35). The net effect of the cisplatin treatment is that the cell undergoes programmed cell death, or apoptosis, killing the cancer cell. Unfortunately, cisplatin is not specific for cancer cells and consequently many normal cells die, causing serious side effects such as nausea, vomiting, and kidney and nerve damage.