35.1 Errors Can Arise in DNA Replication
Errors introduced in the replication process are the simplest source of damage in the double helix. With the addition of each base, there is the possibility that an incorrect base might be incorporated, forming a non-Watson–Crick base pair. These non-Watson–Crick base pairs can locally distort the DNA double helix. Furthermore, such mismatches can be mutagenic; that is, they can result in permanent changes in the DNA sequence. When a double helix containing a non-Watson–Crick base pair is replicated, the two daughter double helices will have different sequences because the mismatched base is very likely to pair with its Watson–Crick partner. Errors other than mismatches include insertions, deletions, and breaks in one or both strands. Such errors are especially damaging because replicative polymerases can stall or even fall off a damaged template entirely. As a consequence, replication of the genome may halt before it is complete.
A variety of mechanisms have evolved to deal with such interruptions, including the recruitment of specialized DNA polymerases, called translesion or error-prone polymerases, to the region of the DNA that contains the lesions (Table 34.1). Because these molecular machines are not as precise as the replicative polymerases, they can replicate DNA across many lesions. However, a drawback to the use of these enzymes is that such polymerases are substantially more error prone than are normal replicative polymerases when replicating normal DNA. Nonetheless, these error-prone polymerases allow the completion of a draft sequence of the damaged area of the genome that can be at least partly repaired by DNA-repair processes. DNA recombination (Section 35.3) provides an additional mechanism for salvaging interruptions in DNA replication.
!clinic! CLINICAL INSIGHT: Some Genetic Diseases Are Caused by the Expansion of Repeats of Three Nucleotides
Some genetic diseases are caused by the presence of DNA sequences that are inherently prone to errors in the course of replication. One particular class of such diseases is characterized by the presence of long tandem arrays of repeats of three nucleotides. An example is Huntington disease, an autosomal dominant neurological disorder with a variable age of onset. The symptoms of Huntington disease include uncontrolled movements (chorea), the loss of cognitive facilities, and personality alterations. The mutated gene in this disease expresses a protein in the brain called huntingtin, which contains a stretch of consecutive glutamine residues. These glutamine residues are encoded by a tandem array of CAG sequences within the gene. In unaffected persons, this array is between 6 and 31 repeats, whereas, in those having the disease, the array is between 36 and 82 repeats or longer. Moreover, the array tends to become longer from one generation to the next. The consequence is a phenomenon called anticipation: the children of an affected parent tend to show symptoms of the disease at an earlier age than did the parent.
The tendency of trinucleotide repeats to expand is explained by the formation of alternative structures in DNA replication (Figure 35.1). Part of the array within a template strand can loop out without disrupting base-pairing outside this region. In replication, DNA polymerase extends this strand through the remainder of the array by a poorly understood mechanism, leading to an increase in the number of copies of the trinucleotide sequence. Friedreich’s ataxia is caused by trinucleotide repeat expansion in the gene encoding frataxin, which disrupts iron–sulfur cluster synthesis and thus mitochondrial function.
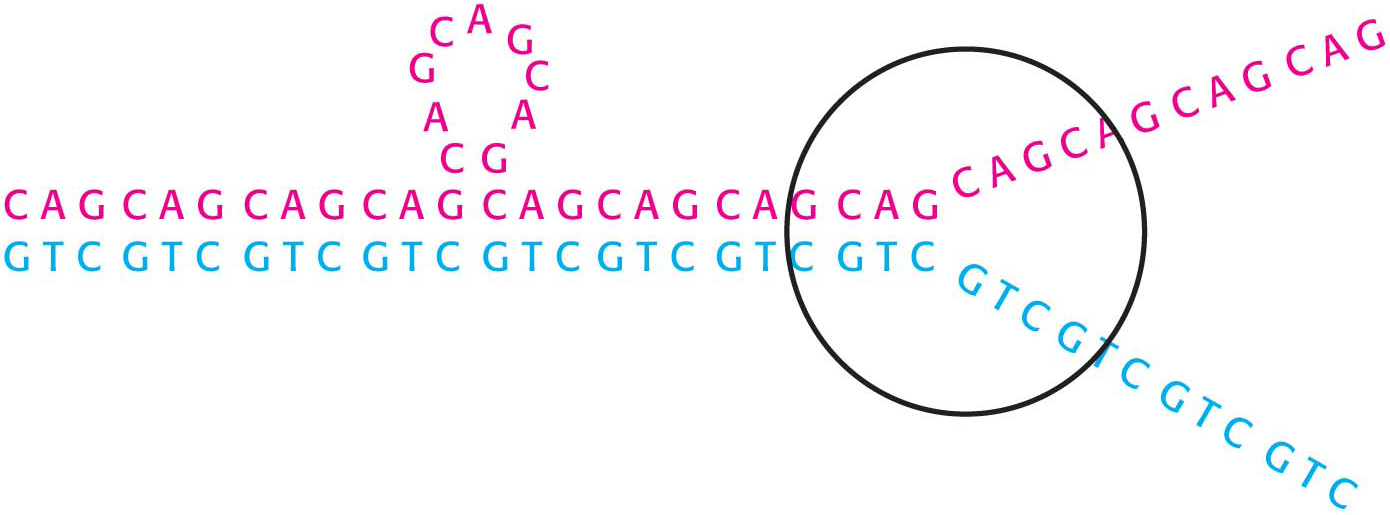
Figure 35.1: Triplet-repeat expansion. Sequences containing tandem arrays of repeated triplet sequences can be expanded to include more repeats by the looping out of some of the repeats before replication. The double helix formed from the red template strand will contain additional sequences encompassing the looped-out region. The circle represents the replication machinery.
A number of other neurological diseases are characterized by expanding arrays of trinucleotide repeats. How do these long stretches of repeated amino acids cause disease? For huntingtin, it appears that the polyglutamine stretches become increasingly prone to aggregate as their length increases; the additional consequences of such aggregation are still under investigation. Thus, Huntington disease and other trinucleotide expansion diseases may be yet other examples of a protein-aggregation disease.
Bases Can Be Damaged by Oxidizing Agents, Alkylating Agents, and Light
The integrity of DNA is constantly under assault, not only from errors in replication, but also by an array of chemical agents. Indeed, an estimated 104 to 106 DNA-damaging events take place in a single human cell in a day. The chemical agents that alter specific bases within DNA after replication is complete are called mutagens and include reactive oxygen species such as hydroxyl radical. For example, hydroxyl radical reacts with guanine to form 8-oxoguanine. 8-Oxoguanine is mutagenic because it often pairs with adenine rather than cytosine in DNA replication (Figure 35.2).
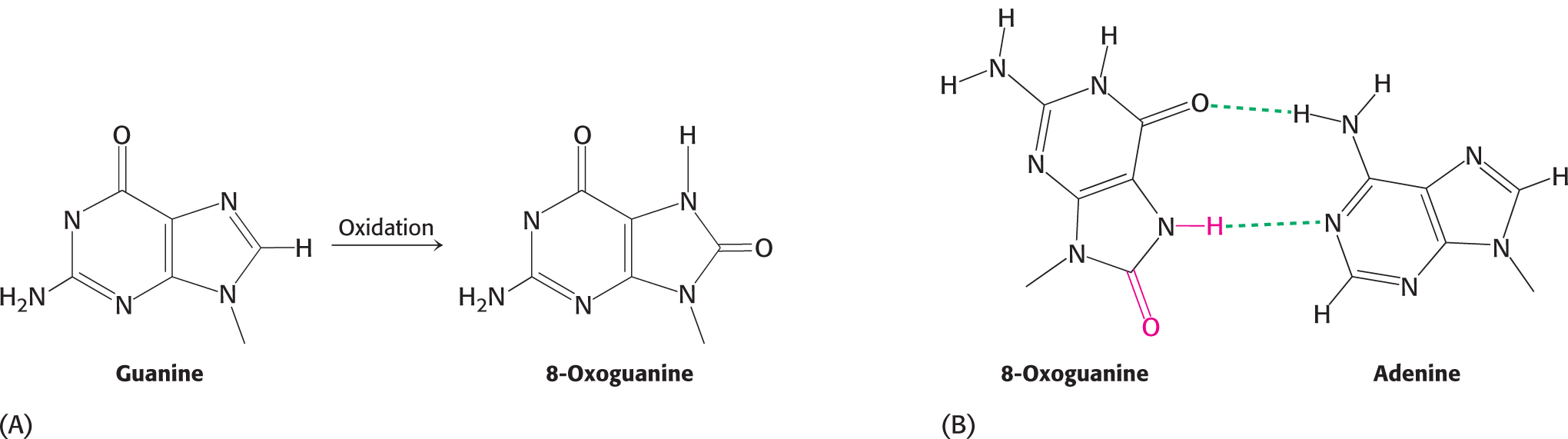
Figure 35.2: Guanine oxidation. (A) Guanine, the base component of dGMP in DNA, can be oxidized to 8-oxoguanine. (B) 8-Oxoguanine can base-pair with adenine.
After replication, one of the replicated helices will have an A–T base pair in place of a G–C base pair. Deamination is another potentially deleterious process. For example, adenine can be deaminated to form hypoxanthine (Figure 35.3). This process is mutagenic because hypoxanthine pairs with cytosine rather than thymine. Guanine and cytosine also can be deaminated to yield bases that pair differently from the parent base.
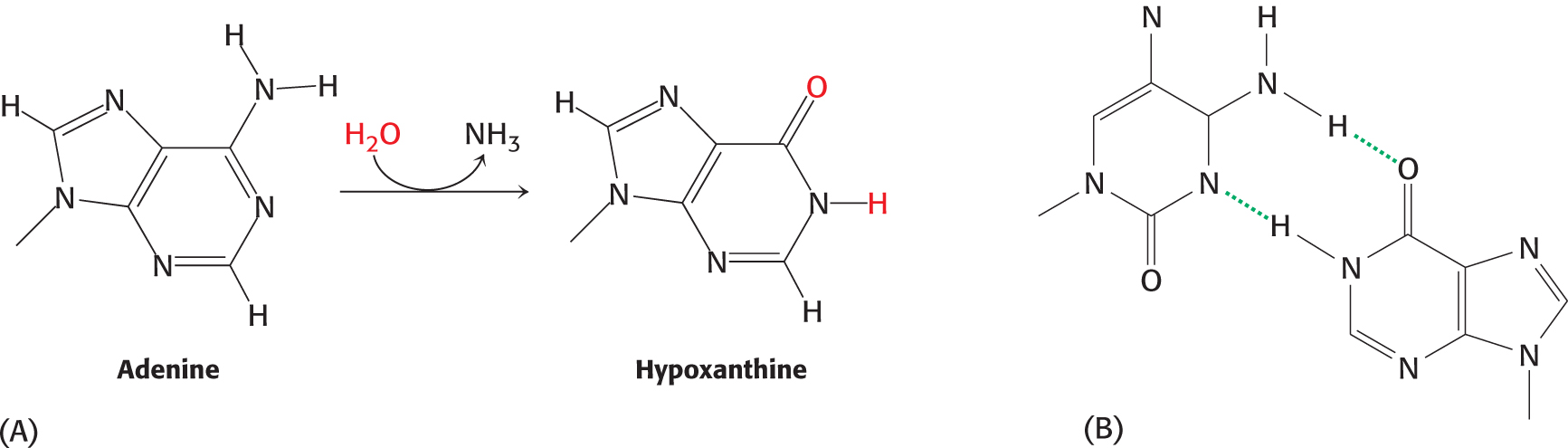
Figure 35.3: Adenine deamination. (A) The base adenine can be deaminated to form hypoxanthine. (B) Hypoxanthine forms base pairs with cytosine in a manner similar to that of guanine, and so the deamination reaction can result in mutation.
DID YOU KNOW?
Epoxide is an oxygen atom bonded to two carbon atoms and is also called a cyclic ether. Because of the strained ring, epoxides are especially reactive.
In addition to oxidation and deamination, nucleotide bases are subject to the addition of a hydrocarbon molecule, a reaction called alkylation. A striking example is aflatoxin B1, a compound produced by molds that grow on peanuts and other foods. A cytochrome P450 enzyme converts this compound into a highly reactive epoxide (Figure 35.4). This agent reacts with the N-7 atom of guanine to form a mutagenic adduct that frequently leads to the conversion of a G–C base pair into a T–A base pair. Polycyclic aromatic hydrocarbons, present in automobile exhaust and cigarette smoke, also are converted into reactive epoxides by a cytochrome P450 enzyme. These epoxides alkylate DNA, accounting in part, for the carcinogenicity of those pollutants.
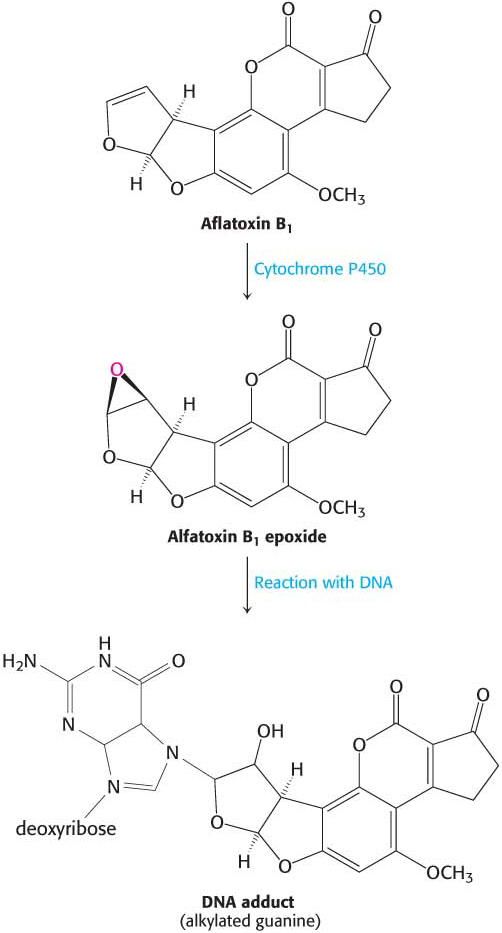
Figure 35.4: Aflatoxin B1 activation. The compound, produced by molds that grow on peanuts and other grains, is activated by a cytochrome P450 enzyme to form a highly reactive species that modifies bases such as guanine in DNA, leading to mutations.
The most pervasive DNA-damaging agent is the ultraviolet component of sunlight (Figure 35.5). Its major effect is to covalently link adjacent pyrimidine residues along a DNA strand (Figure 35.6). Such a pyrimidine dimer cannot fit into a double helix, and so replication and gene expression are blocked until the lesion is removed. The tanning that occurs from exposure to sunlight is due to the formation of melanin pigments. These pigments absorb ultraviolet light and may protect against further DNA damage.
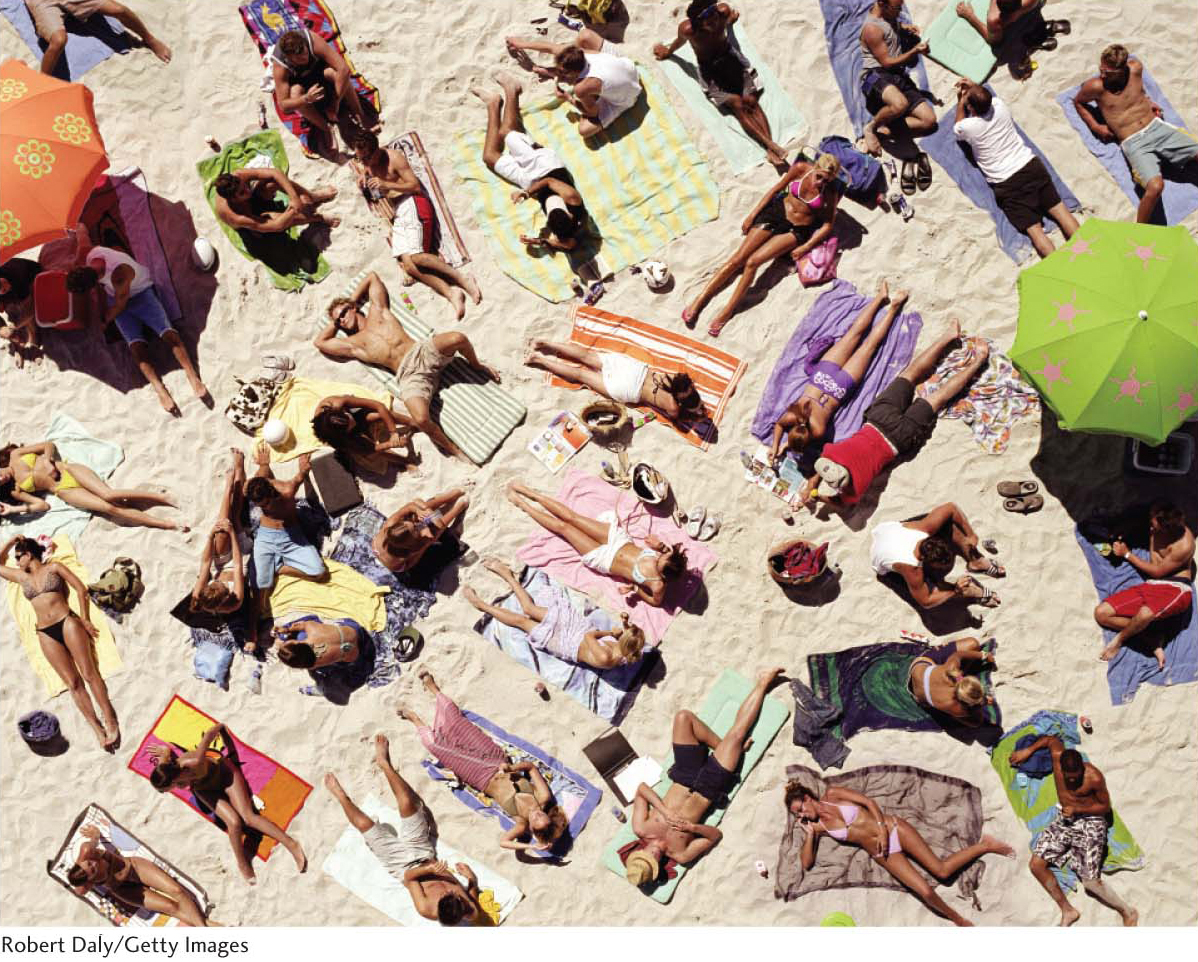
Figure 35.5: Tanning. Ultraviolet light increases skin pigmentation and causes DNA damage.
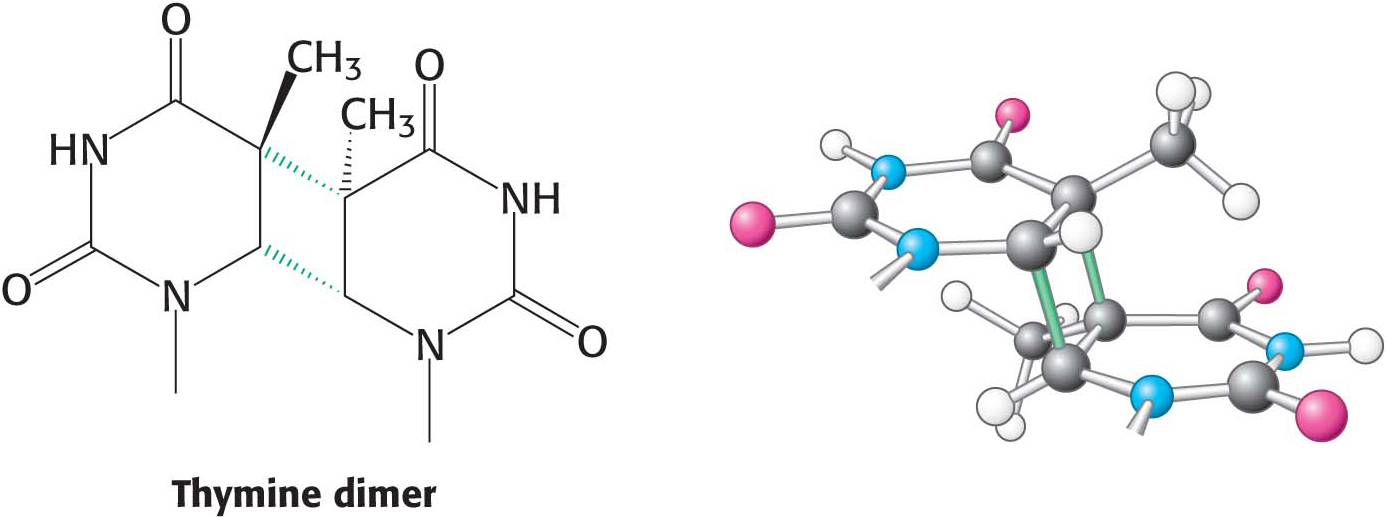
Figure 35.6: A cross-linked dimer of two thymine bases. Ultraviolet light induces cross-links between adjacent pyrimidines along one strand of DNA.
High-energy electromagnetic radiation (ionizing radiation) such as x-rays can damage DNA by producing high concentrations of reactive chemicals. X-ray exposure can also induce several types of DNA damage, including single- and double-stranded breaks in DNA.