36.3 The lac Operon Illustrates the Control of Bacterial Gene Expression
✓ 2 Describe how transcription is controlled in bacteria.
Let us now examine how transcription in bacteria responds to environmental information. An especially clear example is the regulation of genes for the enzymes that catabolize the disaccharide lactose. Bacteria such as E. coli usually rely on glucose as their source of carbon and energy. However, when glucose is scarce, E. coli can use lactose as its carbon source even though this disaccharide does not lie on any major metabolic pathways. An essential enzyme in the metabolism of lactose is β-galactosidase, which hydrolyzes lactose into galactose and glucose. These products are then metabolized by pathways discussed in Chapter 16.
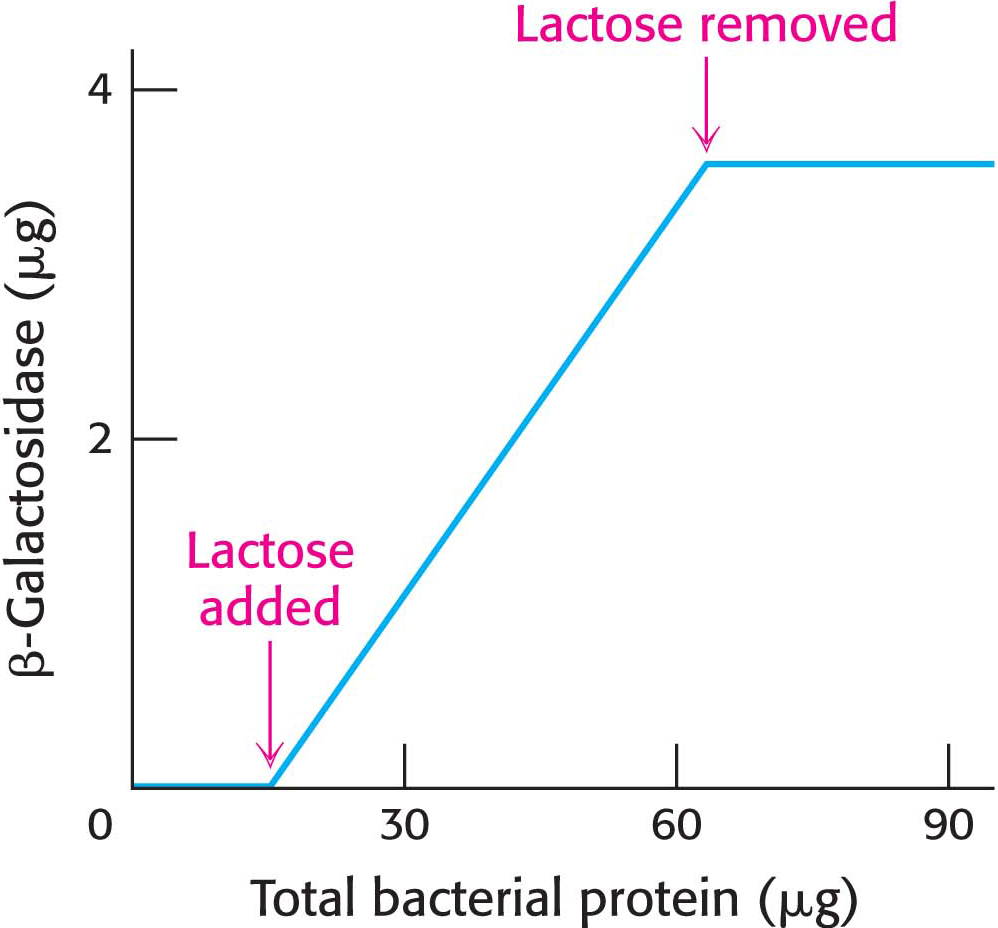
Figure 36.16: β-Galactosidase induction. The addition of lactose to an E. coli culture causes the production of β-galactosidase to increase from very low amounts to much larger amounts. The increase in the amount of enzyme parallels the increase in the number of cells in the growing culture. β-Galactosidase constitutes 6.6% of the total protein synthesized in the presence of lactose.
An E. coli cell growing on a carbon source such as glucose or glycerol contains fewer than 10 molecules of β-galactosidase. In contrast, the same cell will contain several thousand molecules of the enzyme when grown on lactose (Figure 36.16).
Interestingly, two other proteins are synthesized whenever β-galactosidase is synthesized—namely, galactoside permease and thiogalactoside transacetylase. The permease is required for the transport of lactose across the bacterial cell membrane. The transacetylase is not essential for lactose metabolism but may play a role in the detoxification of compounds that also may be transported by the permease. The expression levels of a set of enzymes that all contribute to adaptation to a specific change in the environment change together. Such a coordinated unit of gene expression is called an operon.
An Operon Consists of Regulatory Elements and Protein-Encoding Genes
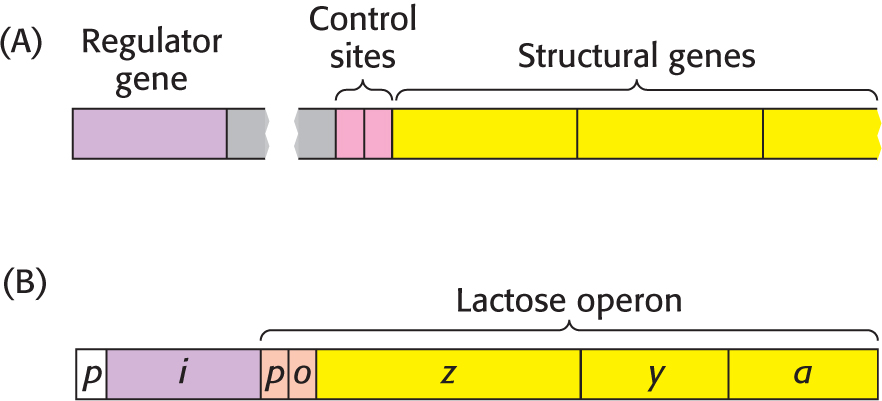
Figure 36.17: Operons. (A) The general structure of an operon. (B) The structure of the lactose operon. In addition to the promoter, p, in the operon, a second promoter is present in front of the regulator gene, i, to drive the synthesis of the regulator.
The parallel regulation of β-galactosidase, the permease, and the transacetylase suggests that the expression of genes encoding these enzymes is controlled by a single mechanism (Figure 36.17). The DNA components of the regulatory system are a regulator gene, an operator site, and a set of structural genes that, in the galactosidase example, encode the three enzymes. The regulator gene encodes a repressor protein that binds to the operator site. As the name suggests, binding of the repressor to the operator represses transcription of the structural genes. The operator and its associated structural genes constitute the operon.
For the lactose (lac) operon, the gene encoding the repressor is designated i, the operator site is o, and the structural genes for β-galactosidase, the permease, and the transacetylase are called z, y, and a, respectively. The operon also contains a promoter site (denoted by p), which directs the RNA polymerase to the correct transcription-initiation site. The z, y, and a genes are transcribed to yield a single mRNA molecule that encodes all three proteins. An mRNA molecule encoding more than one protein is known as a polygenic or polycistronic transcript.
How does the lac repressor inhibit the expression of the lac operon? In the absence of lactose, the repressor binds very tightly and rapidly to the operator, blocking the bound RNA polymerase from using the DNA as a template. The biochemical rationale is that, in the absence of lactose, there is no need to transcribe the genes that encode enzymes required for lactose degradation.
Ligand Binding Can Induce Structural Changes in Regulatory Proteins
How is the repressor removed from the operator? This question is tantamount to asking what signal triggers the expression of the lac operon. A signal molecule called an inducer binds to the lac repressor, causing a structural change in the repressor that greatly reduces the affinity of the repressor for the operator DNA. The repressor–inducer complex leaves the DNA and allows transcription of the operon (Figure 36.18).
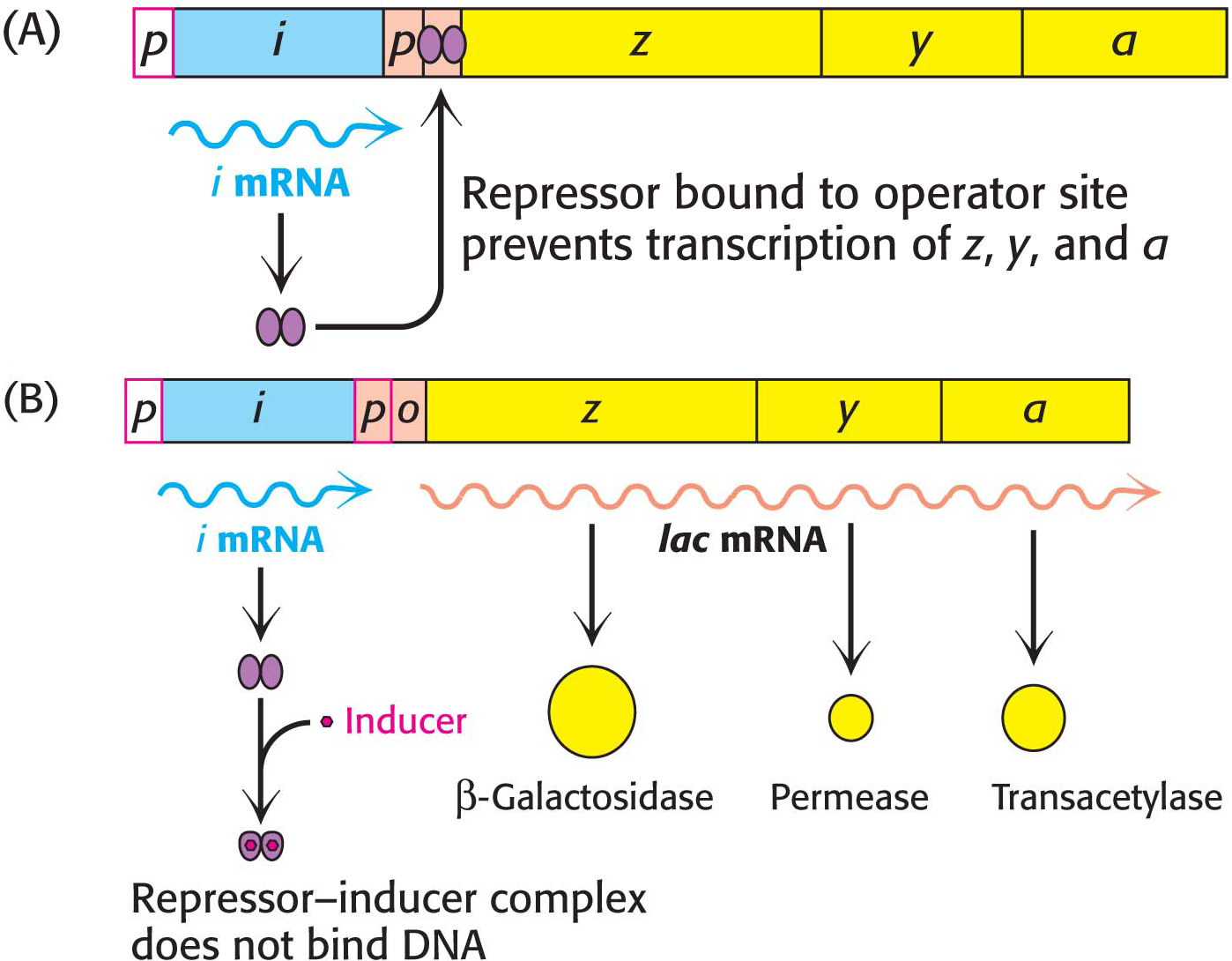
Figure 36.18: The induction of the lac operon. (A) In the absence of lactose, the lac repressor binds DNA and represses transcription from the lac operon. (B) Allolactose or another inducer binds to the lac repressor, leading to its dissociation from DNA and to the production of lac mRNA.
What is the inducer? Intuitively, we would expect it to be lactose itself. Interestingly, however, it is not lactose that announces its own presence; rather, it is allolactose, a combination of galactose and glucose with an α-1,6 rather than an α-1,4 linkage, as in lactose. Allolactose is a side product of the β-galactosidase reaction produced at low levels by the few molecules of β-galactosidase that are present before induction. Many other gene-regulatory networks function in ways analogous to those of the lac operon.
Transcription Can Be Stimulated by Proteins That Contact RNA Polymerase
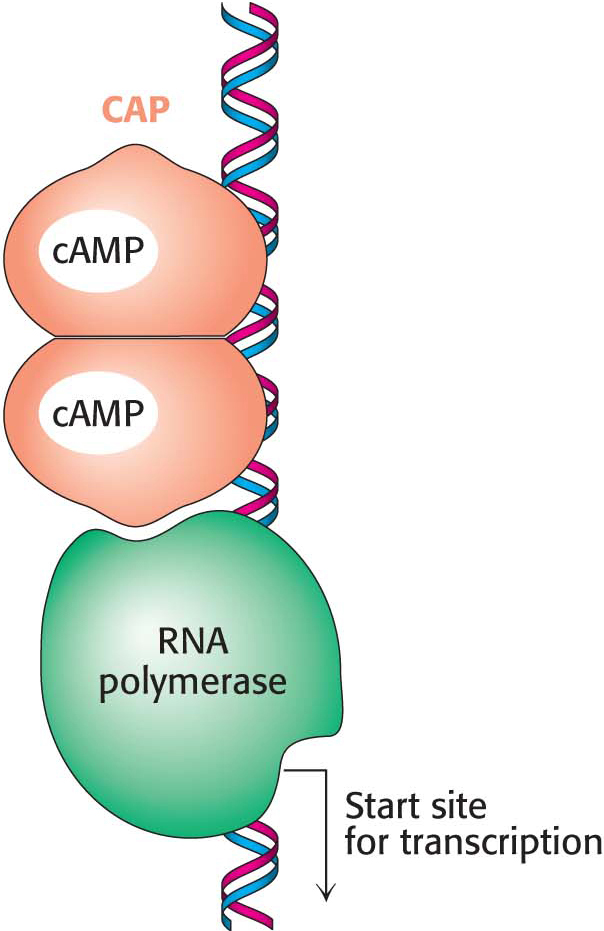
Figure 36.19: The binding site for catabolite activator protein (CAP). This protein binds as a dimer to an inverted repeat that is at the position −61 relative to the start site of transcription. The CAP-binding site on DNA is adjacent to the position at which RNA polymerase binds.
The lac repressor functions by inhibiting transcription until lactose is present. There are also sequence-specific DNA-binding proteins that stimulate the transcription of the lac operon when glucose is in short supply. One particularly well-studied example is the catabolite activator protein (CAP), which is also known as the cyclic AMP (cAMP) response protein (CRP). When bound to cAMP, CAP stimulates the transcription of lactose genes. When the concentration of glucose decreases, the concentration of cAMP increases, thus activating the lac operon to help E. coli metabolize lactose for fuel.
Within the lac operon, the CAP–cAMP complex binds to a site that is centered near position −61 relative to the start site for transcription. Binding stimulates the initiation of transcription by approximately 50-fold by recruiting RNA polymerase to promoters to which CAP is bound (Figure 36.19). The CAP–cAMP complex forms protein–protein contacts with the RNA polymerase that increases transcription initiation. Thus, in regard to the lac operon, gene expression is maximal when the binding of allolactose relieves the inhibition by the lac repressor, and the CAP–cAMP complex stimulates the binding of RNA polymerase. Glucose, on the other hand, inhibits the expression of the lac operon in a process called catabolite repression. An increase in cellular glucose concentration leads to a decrease in the intracellular concentration of cAMP.
!quickquiz! QUICK QUIZ 2
Describe how allolactose and cAMP combine to regulate the lac operon.
How is the level of cAMP controlled in bacteria? Enzyme IIA (EIIA) is phosphorylated at the expense of the glycolytic intermediate phosphoenolpyruvate. Phosphorylated EIIA then transfers a phosphate to a glucose molecule, generating glucose 6-phosphate. However, if glucose is absent, phosphorylated EIIA activates adenylate cyclase, leading to an increase in cAMP and enhanced transcription of the lac operon.
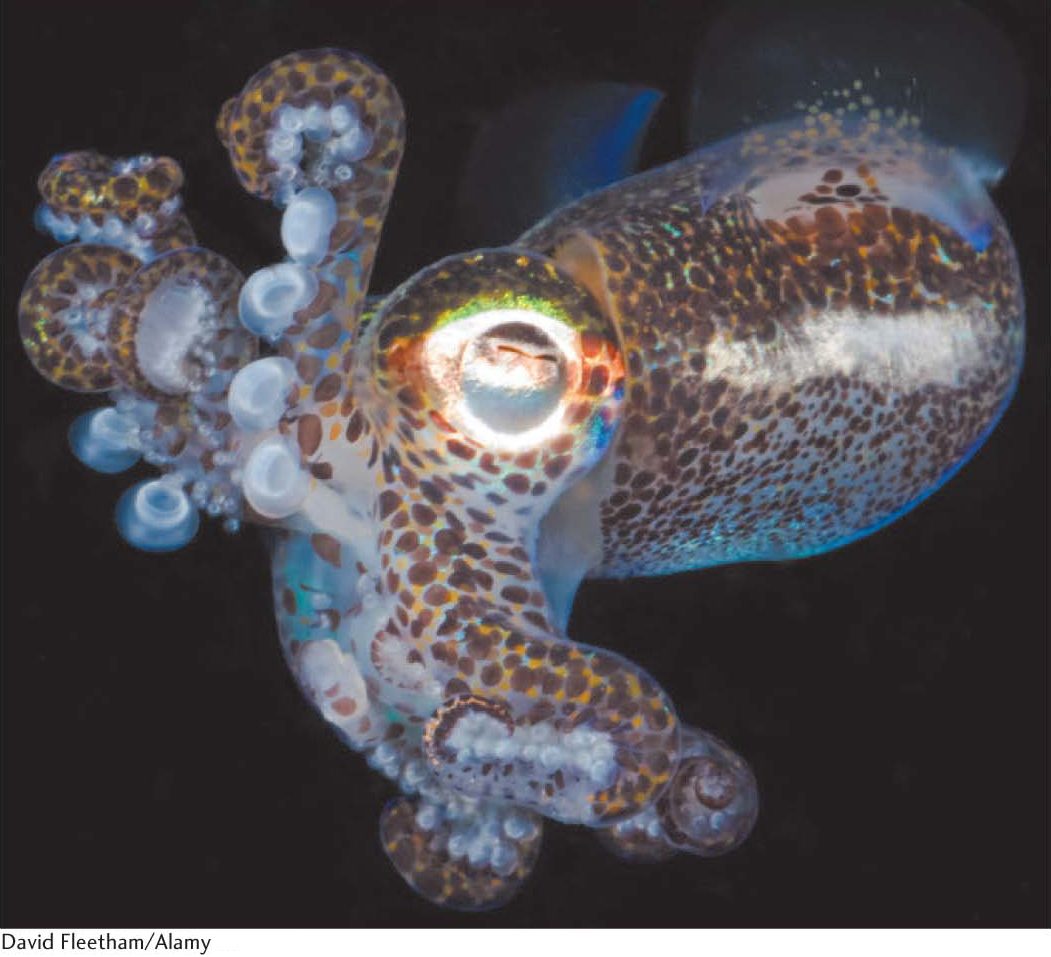
Figure 36.20: Bobtail squid. The bobtail squid, only 1 to 8 cm in length, inhabits the coastal waters of the Pacific and provides safe haven for the bacterium Vibrio fischeri.
!clinic!!bio! CLINICAL AND BIOLOGICAL INSIGHT: Many Bacterial Cells Release Chemical Signals that Regulate Gene Expression in Other Cells
Bacterial cells have been traditionally viewed as solitary single cells. However, it is becoming increasingly clear that, in many circumstances, bacterial cells live in complex communities, interacting with other cells of their own species and of different species. These social interactions change the patterns of gene expression within the cells.
A prominent type of interaction is called quorum sensing. This phenomenon was discovered in Vibrio fischeri, a species of bacterium that can live inside a specialized light organ in the bobtail squid (Figure 36.20). The bobtail squid inhabits shallow waters and hunts at night. On moonlit nights, the squid would be visible to predators prowling below it. The squid adjusts the light emitted by the light organ to match the background, thus becoming less visible to predators. In this symbiotic relation, the bacteria produce the enzyme luciferase, which generates bioluminescence, thereby providing protection for the squid. In return, the bacteria have a protected place to live and reproduce. Interestingly, when these bacteria are grown in culture at low density, they are not bioluminescent. However, when the cell density reaches a critical level, the gene for luciferase is expressed and the cells bioluminesce. How do these cells sense the density of their population?
Cells of V. fischeri release an autoinducer into their environment and other V. fischeri cells take up the chemical. After the inducer concentration inside the cell has increased to an appropriate level, the inducer binds to a regulatory protein. The complex of inducer–regulatory protein then binds to promoter sites, increasing the rate of transcription of the operon that encodes the luciferase gene as well as the gene for the autoinducer. Because each cell produces only a small amount of the autoinducer, this regulatory system allows each V. fischeri cell to determine the density of the V. fischeri population in its environment—hence, the term quorum sensing for this process.
Quorum sensing appears to play a major role in the formation of bacterial communities with particular species compositions. Many species of bacteria can be found in specialized structures termed biofilms that can form on surfaces. Some genes controlled by quorum-sensing mechanisms promote the formation of specific molecules, which include proteins, polysaccharides, and nucleic acids, that serve as a matrix for the biofilm. Biofilms are of considerable medical importance. Organisms within them are more than 1000-fold more resistant to antibiotics and more able to evade the host immune response than free-living bacteria. Patients implanted with prosthetic devices, including simple bladder catheters, are especially at risk for biofilm formation. Dental plaque and shower scum are common examples of biofilms.
Some Messenger RNAs Directly Sense Metabolite Concentrations
A newly discovered control mechanism for the regulation of gene expression depends on the remarkable ability of some mRNA molecules to form special secondary structures, some of which are capable of directly binding small molecules. These structures are termed riboswitches. Consider a riboswitch-containing RNA that encodes the genes that participate in the biosynthesis of riboflavin in bacteria. As synthesis is taking place, the RNA can adopt two alternative structures—one that is capable of binding flavin mononucleotide (FMN), a key intermediate in riboflavin synthesis, or one that is not. The binding of FMN traps the RNA transcript in a conformation that favors the termination of further RNA synthesis, preventing the production of functional mRNA. This strategy makes good biochemical sense: if the precursor is present, it is not necessary to make the enzymes that synthesize the precursor. However, when FMN is absent, an alternative conformation forms that allows the production of the full-length mRNA. Riboswitches serve as a vivid illustration of how RNAs are capable of forming elaborate, functional structures, though we tend to depict them as simple lines in the absence of specific information.