38.4 RNA Can Function as a Catalyst
RNAs are a surprisingly versatile class of molecules. As we have seen, splicing is catalyzed largely by RNA molecules, with proteins playing a secondary role. As we shall see in Chapter 40, the RNA component of ribosomes is the catalyst that carries out protein synthesis. The discovery of catalytic RNA revolutionized the concept of biological catalysis.
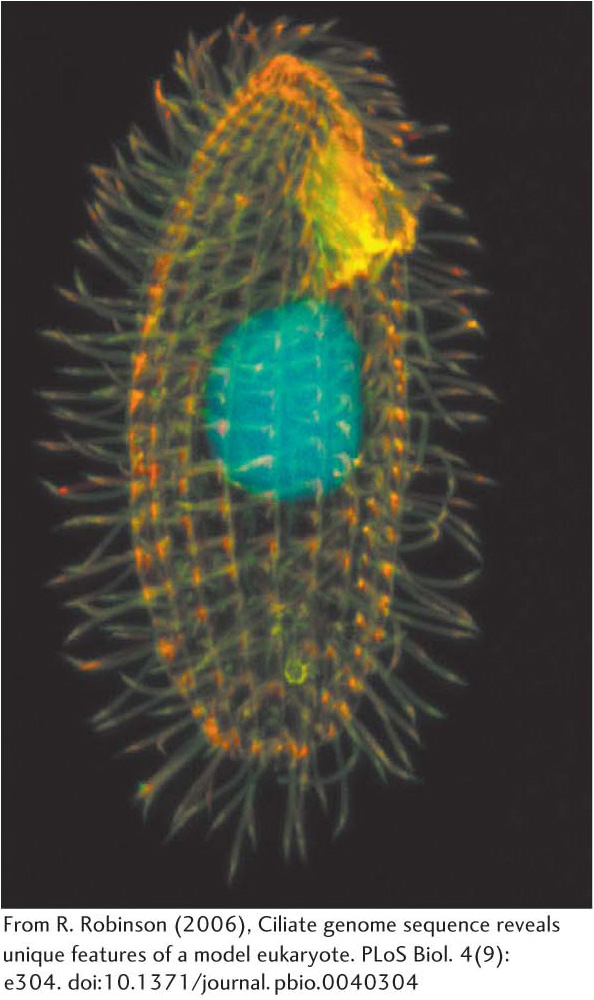
Figure 38.13: Tetrahymena.
The versatility of RNA first became clear from observations of the processing of ribosomal RNA in a single-cell eukaryote, Tetrahymena—a ciliated protozoan (Figure 38.13). In this reaction, a 414-nucleotide intron is removed from a precursor to yield the mature rRNA molecule (Figure 38.14). In an elegant series of studies of this splicing reaction, Thomas Cech and his coworkers established that the RNA spliced itself to precisely excise the intron in the absence of protein. Indeed, the RNA alone is catalytic under certain conditions and thus can function as an enzyme. Catalytic RNAs are called ribozymes. Catalytic biomolecules, formerly believed to be limited to proteins, now include certain RNA molecules. More than 1500 similar introns have since been found in species as widely dispersed as bacteria and eukaryotes, though not in vertebrates. Collectively, they are referred to as group I introns.
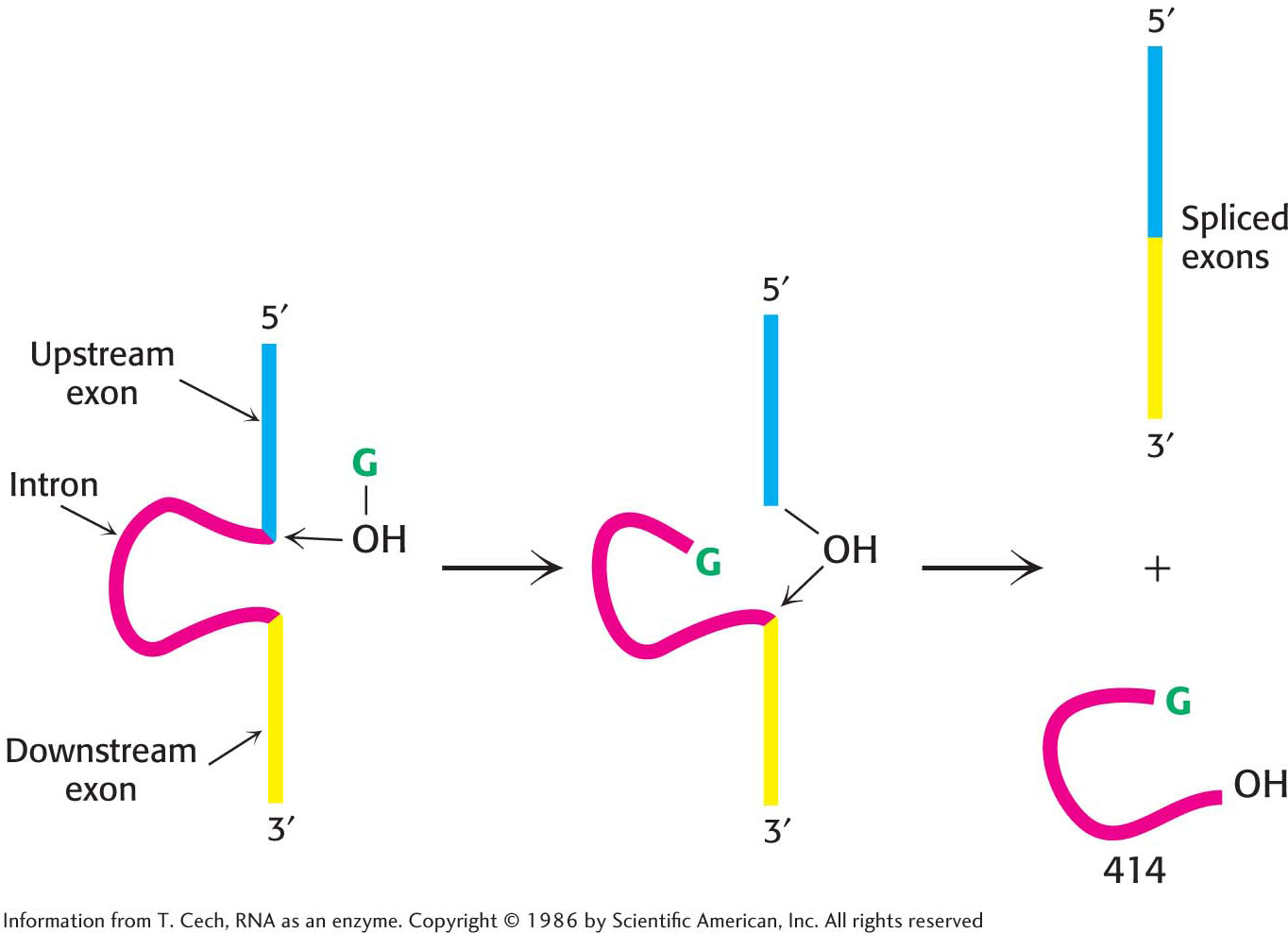
Figure 38.14: Self-splicing. A ribosomal RNA precursor from Tetrahymena splices itself in the presence of a guanosine cofactor (G, shown in green). An intron (red) is released in the first splicing reaction. This intron then splices itself twice again to produce a linear RNA.
The self-splicing reaction in the group I intron requires an added guanosine or guanine nucleotide. Guanosine serves not as an energy source but as an attacking group that becomes transiently incorporated into the RNA (Figure 38.14). Guanosine associates with the RNA and then attacks the 5′ splice site to form a phosphodiester linkage with the 5′ end of the intron, generating a 3′-OH group at the end of the upstream exon. This newly generated 3′-OH group then attacks the 3′ splice site, joining the two exons and releasing the intron. Self-splicing depends on the structural integrity of the RNA precursor. This molecule, like many RNAs, has a folded structure formed by many double-helical stems and loops (Figure 38.15), with a well-defined pocket for binding the guanosine.
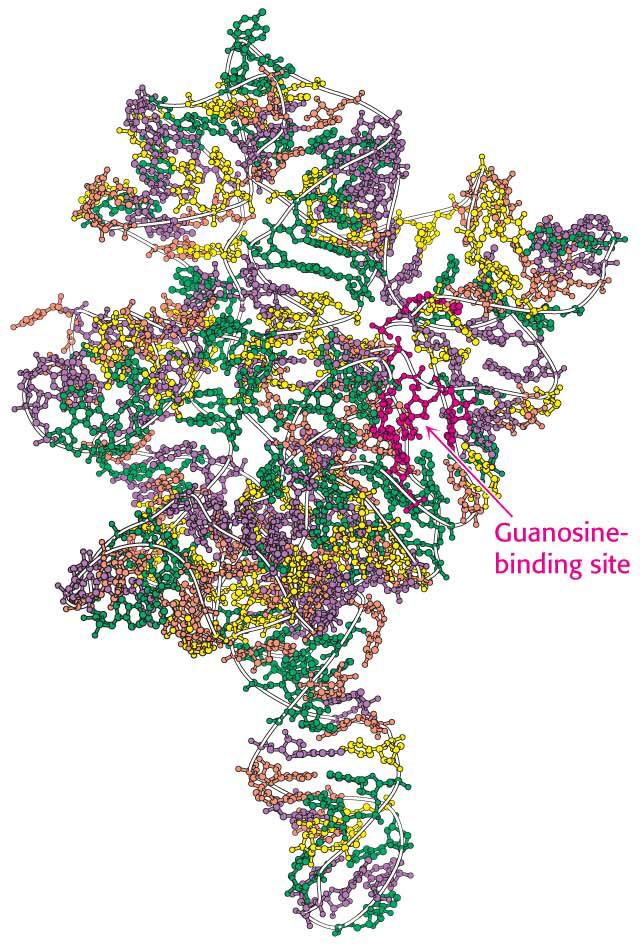
Figure 38.15:
The structure of a self-splicing intron. The structure of a large fragment of the self-splicing intron from Tetrahymena reveals a complex folding pattern of helices and loops. Bases are shown in green, A; yellow, C; purple, G; and orange, U
Messenger RNA precursors in the mitochondria of yeast and fungi also undergo self-splicing, as do some RNA precursors in the chloroplasts of unicellular organisms such as Chlamydomonas. Self-splicing reactions can be classified according to the nature of the unit that attacks the upstream splice site. Group I self-splicing is mediated by a guanosine cofactor, as in Tetrahymena. The attacking moiety in group II splicing is the 2′-OH group of a specific adenylate of the intron.