4.2 Secondary Structure: Polypeptide Chains Can Fold into Regular Structures
Can a polypeptide chain fold into a regularly repeating structure? In 1951, Linus Pauling and Robert Corey proposed that certain polypeptide chains have the ability to fold into two periodic structures called the α helix (alpha helix) and the β pleated sheet (beta pleated sheet). Subsequently, other structures such as turns and loops were identified. Alpha helices, β pleated sheets, and turns are formed by a regular pattern of hydrogen bonds between the peptide NH and CO groups of amino acids that are often near one another in the linear sequence, or primary structure. Such regular folded segments are called secondary structure.
The Alpha Helix Is a Coiled Structure Stabilized by Intrachain Hydrogen Bonds
DID YOU KNOW?
Screw sense refers to the direction in which a helical structure rotates with respect to its axis. If viewed down the axis of a helix (N terminus to C terminus), the chain turns in a clockwise direction; it has a right-handed screw sense. If turning is counterclockwise, the screw sense is left-handed.
The first of Pauling and Corey’s proposed secondary structures was the α helix, a rodlike structure with a tightly coiled backbone. The side chains of the amino acids composing the structure extend outward in a helical array (Figure 4.11). The α helix is stabilized by hydrogen bonds between the NH and CO groups of the main chain. The CO group of each amino acid forms a hydrogen bond with the NH group of the amino acid that is situated four residues ahead in the sequence (Figure 4.12). Thus, except for amino acids near the ends of an α helix, all the main-chain CO and NH groups are hydrogen bonded. Each residue is related to the next one by a rise, also called translation, of 1.5 Å along the helix axis and a rotation of 100 degrees, which gives 3.6 amino acid residues per turn of helix. Thus, amino acids spaced three and four apart in the sequence are spatially quite close to one another in an α helix. In contrast, amino acids spaced two apart in the sequence are situated on opposite sides of the helix and so are unlikely to make contact. The pitch of the α helix is the length of one complete turn along the helix axis and is equal to the product of the translation (1.5 Å) and the number of residues per turn (3.6), or 5.4 Å. The screw sense of α helix can be right-handed (clockwise) or left-handed (counterclockwise). Right-handed helices are energetically more favorable because there are fewer steric clashes between the side chains and the backbone. Essentially all α helices found in proteins are right-handed. In schematic representations of proteins, α helices are depicted as twisted ribbons or rods (Figure 4.13).
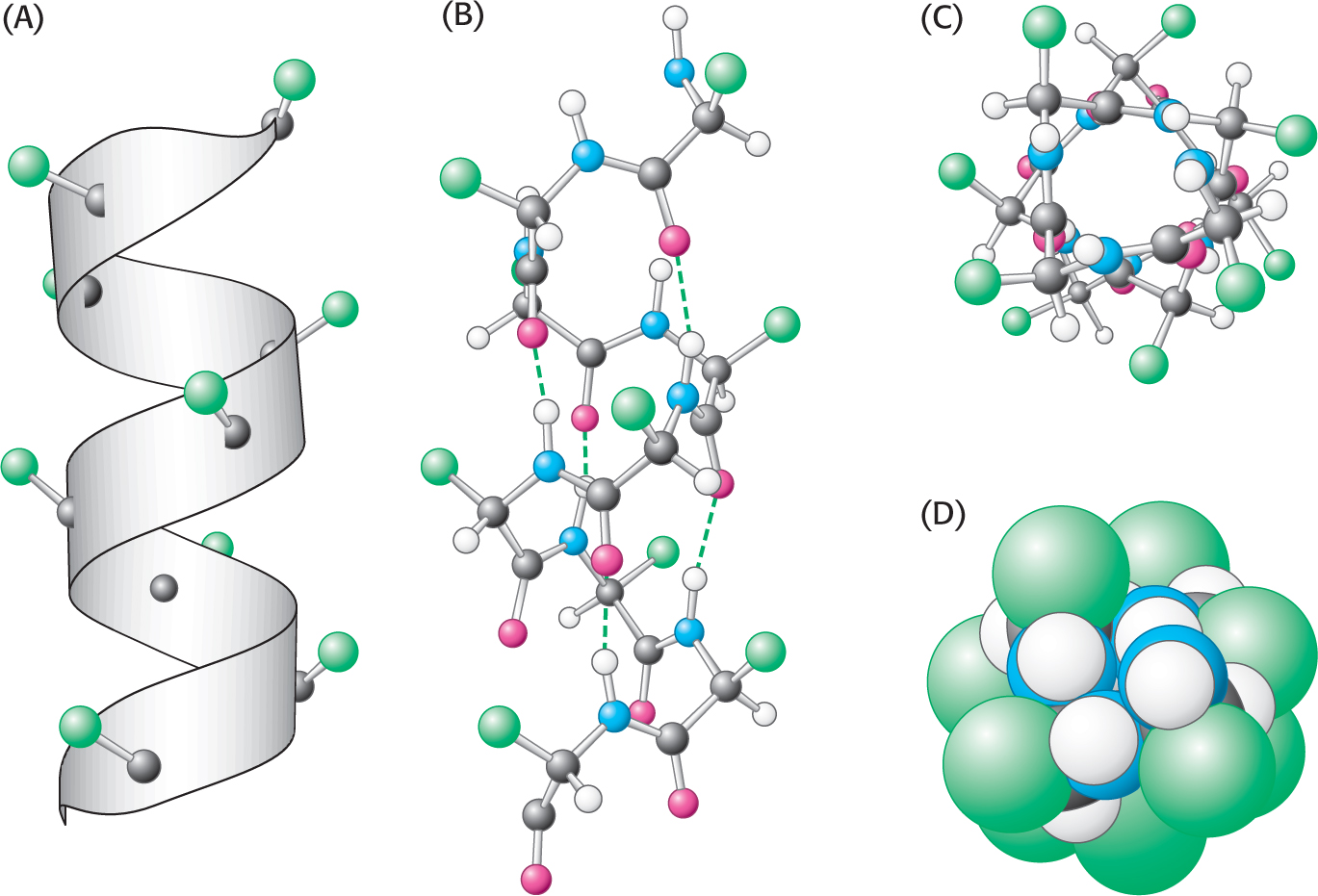
Figure 4.11: The structure of the α helix. (A) A ribbon depiction shows the α-carbon atoms and side chains (green). (B) A side view of a ball-and-stick version depicts the hydrogen bonds (dashed lines) between NH and CO groups. (C) An end view shows the coiled backbone as the inside of the helix and the side chains (green) projecting outward. (D) A space-filling view of part C shows the tightly packed interior core of the helix.
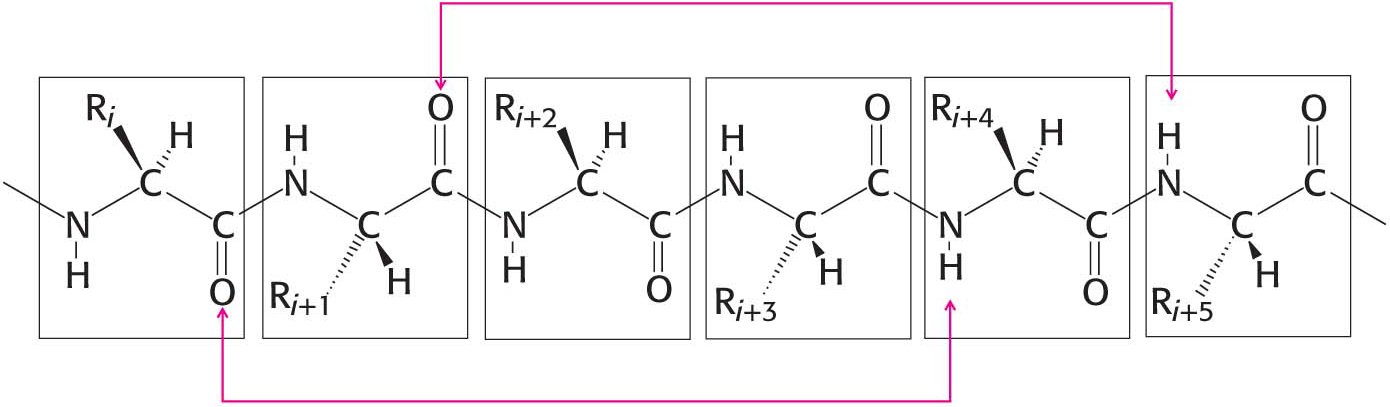
Figure 4.12: The hydrogen-bonding scheme for an α helix. In the α helix, the CO group of residue i forms a hydrogen bond with the NH group of residue i + 4.
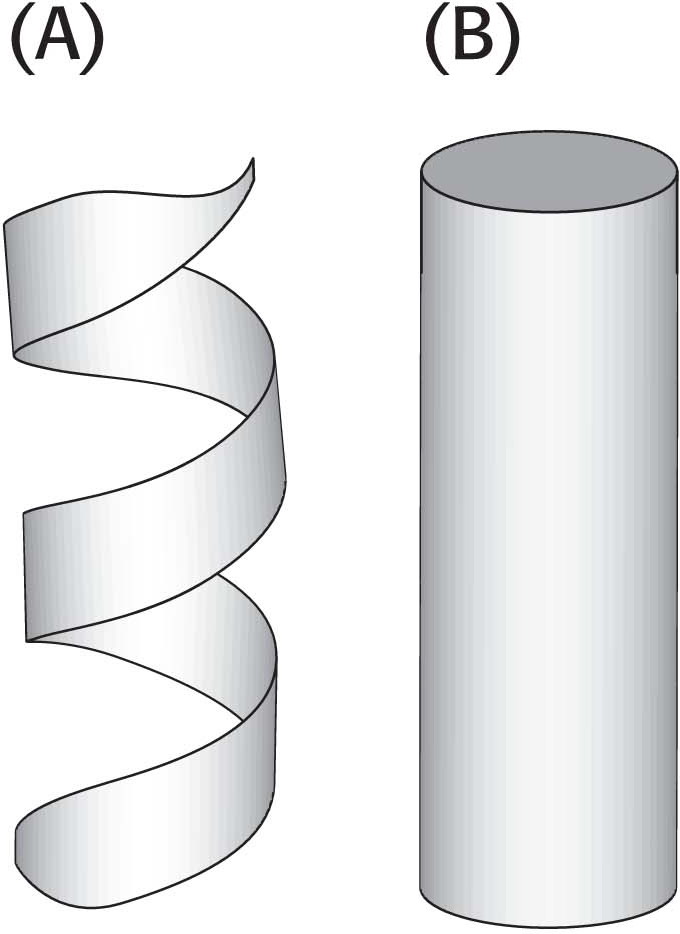
Figure 4.13: Schematic views of α helices. (A) a ribbon depiction. (B) a cylindrical depiction.
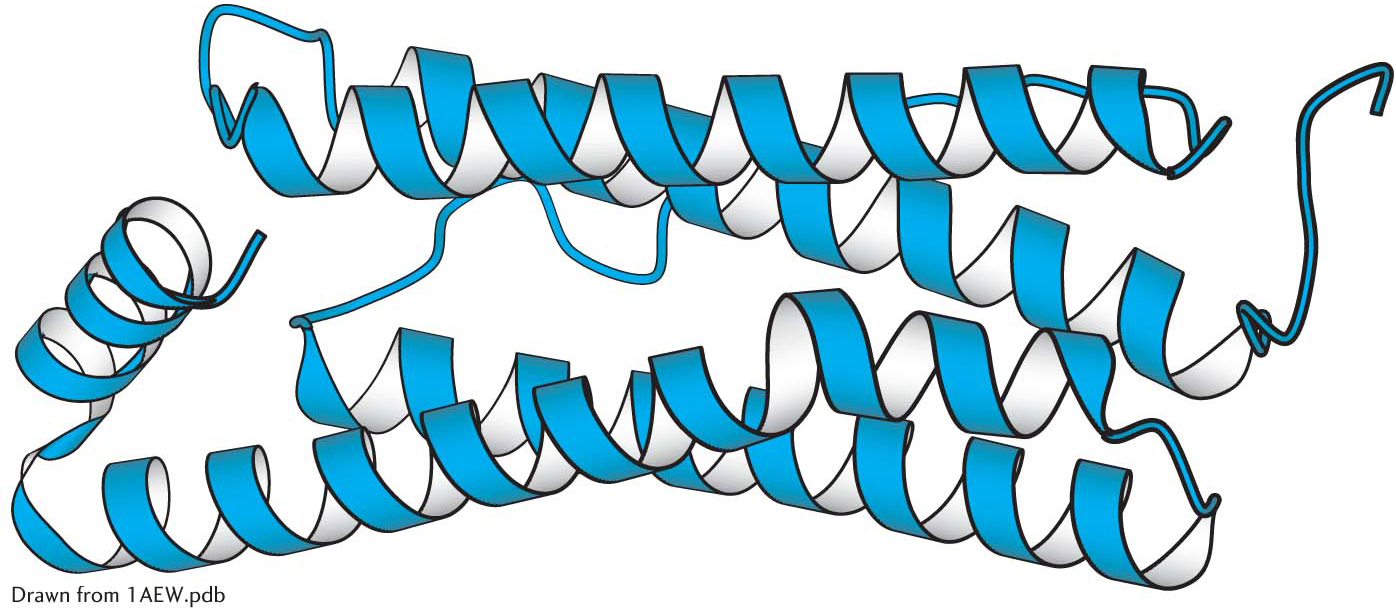
Figure 4.14:
A largely α-helical protein. Ferritin, an iron-storage protein, is built from a bundle of α helices.
Not all amino acids can be readily accommodated in an α helix. Branching at the β-carbon atom, as in valine, threonine, and isoleucine, tends to destabilize α helices because of steric clashes. Serine, aspartate, and asparagine also tend to disrupt α helices because their side chains contain hydrogen-bond donors or acceptors in close proximity to the main chain, where they compete for main-chain NH and CO groups. Proline also is an α helix breaker because it lacks an NH group and because its ring structure prevents it from assuming the ϕ value to fit into an α helix.
The α-helical content of proteins ranges widely, from none to almost 100%. For example, about 75% of the residues in ferritin, an iron-storage protein, are in α helices (Figure 4.14). Indeed, about 25% of all soluble proteins are composed of α helices connected by loops and turns of the polypeptide chain. Single α helices are usually less than 45 Å long. Many proteins that span biological membranes also contain α helices.
Beta Sheets Are Stabilized by Hydrogen Bonding Between Polypeptide Strands
Pauling and Corey named their other proposed periodic structural motif the β pleated sheet (β because it was the second structure that they elucidated). The β pleated sheet (more simply, the β sheet) differs markedly from the rodlike α helix in appearance and bond structure.
Instead of a single polypeptide strand, the β sheet is composed of two or more polypeptide chains called β strands. A β strand is almost fully extended rather than being tightly coiled as in the α helix. The distance between adjacent amino acids along a β strand is approximately 3.5 Å, in contrast with a distance of 1.5 Å along an α helix. The side chains of adjacent amino acids point in opposite directions (Figure 4.15).
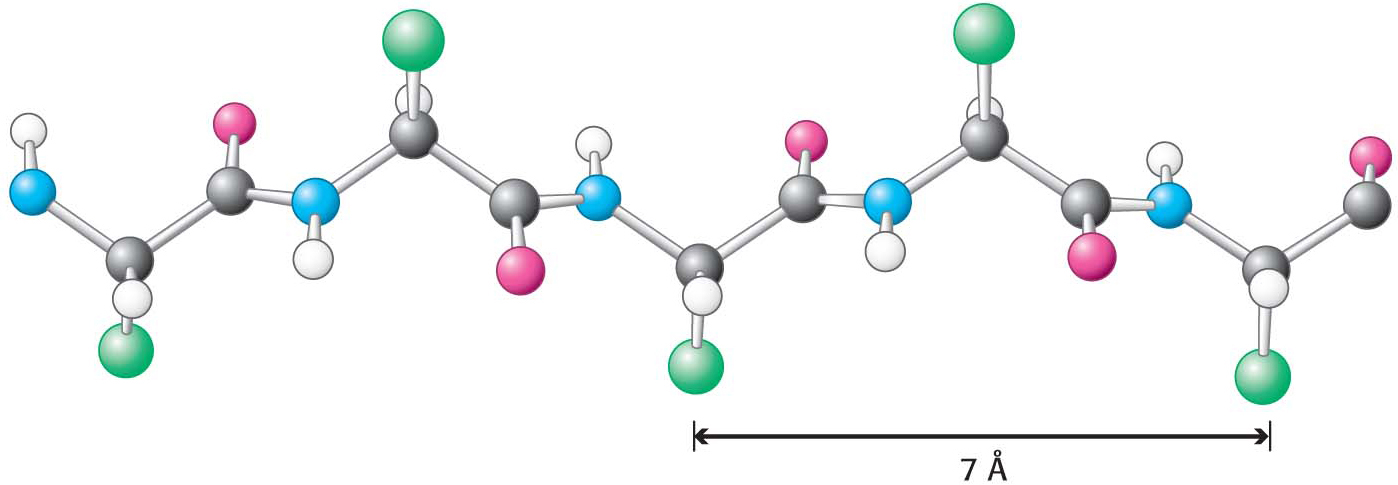
Figure 4.15: The structure of a β strand. The side chains (green) are alternately above and below the plane of the strand. The bar shows the distance between two residues.
A β sheet is formed by linking two or more β strands lying next to one another through hydrogen bonds. Adjacent chains in a β sheet can run in opposite directions (antiparallel β sheet) or in the same direction (parallel β sheet), as shown in Figure 4.16. Many strands, typically 4 or 5 but as many as 10 or more, can come together in a β sheet. Such β sheets can be purely antiparallel, purely parallel, or mixed (Figure 4.17). Unlike α helices, β sheets can consist of sections of a polypeptide that are not near one another. That is, in two β strands that lie next to each other, the last amino acid of one strand and the first amino acid of the adjacent strand are not necessarily neighbors in the amino acid sequence.
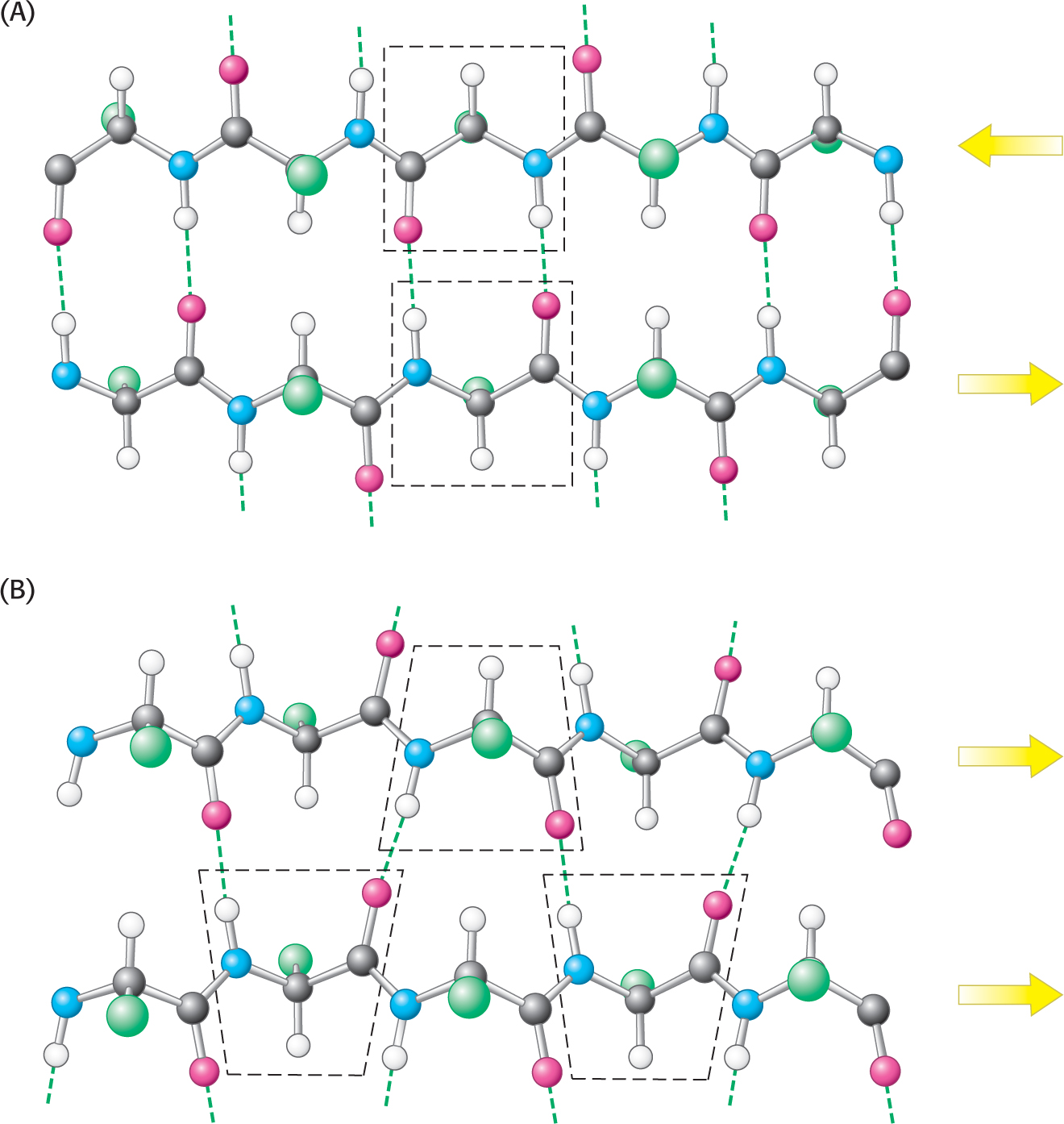
Figure 4.16: Antiparallel and parallel β sheets. (A) Adjacent β strands run in opposite directions. Hydrogen bonds (green dashes) between NH and CO groups connect each amino acid to a single amino acid on an adjacent strand, stabilizing the structure. (B) Adjacent β strands run in the same direction. Hydrogen bonds connect each amino acid on one strand with two different amino acids on the adjacent strand.
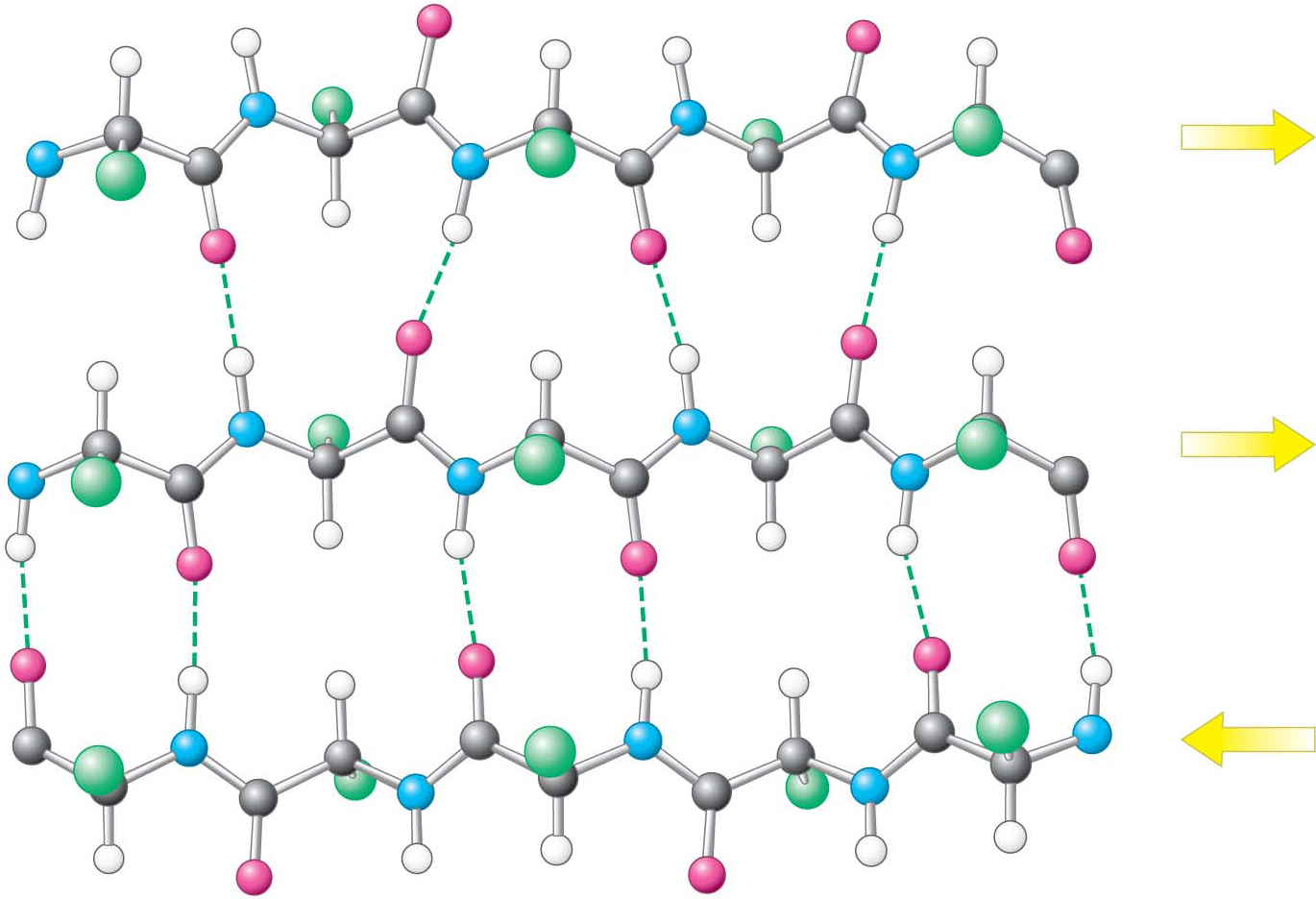
Figure 4.17: The structure of a mixed β sheet.
In schematic representations, β strands are usually depicted by broad arrows pointing in the direction of the carboxyl-terminal end to indicate the type of β sheet formed—parallel or antiparallel. Beta sheets can be almost flat, but most adopt a somewhat twisted shape (Figure 4.18). The β sheet is an important structural element in many proteins. For example, fatty-acid-binding proteins, which are important for lipid metabolism, are built almost entirely from β sheets (Figure 4.19).
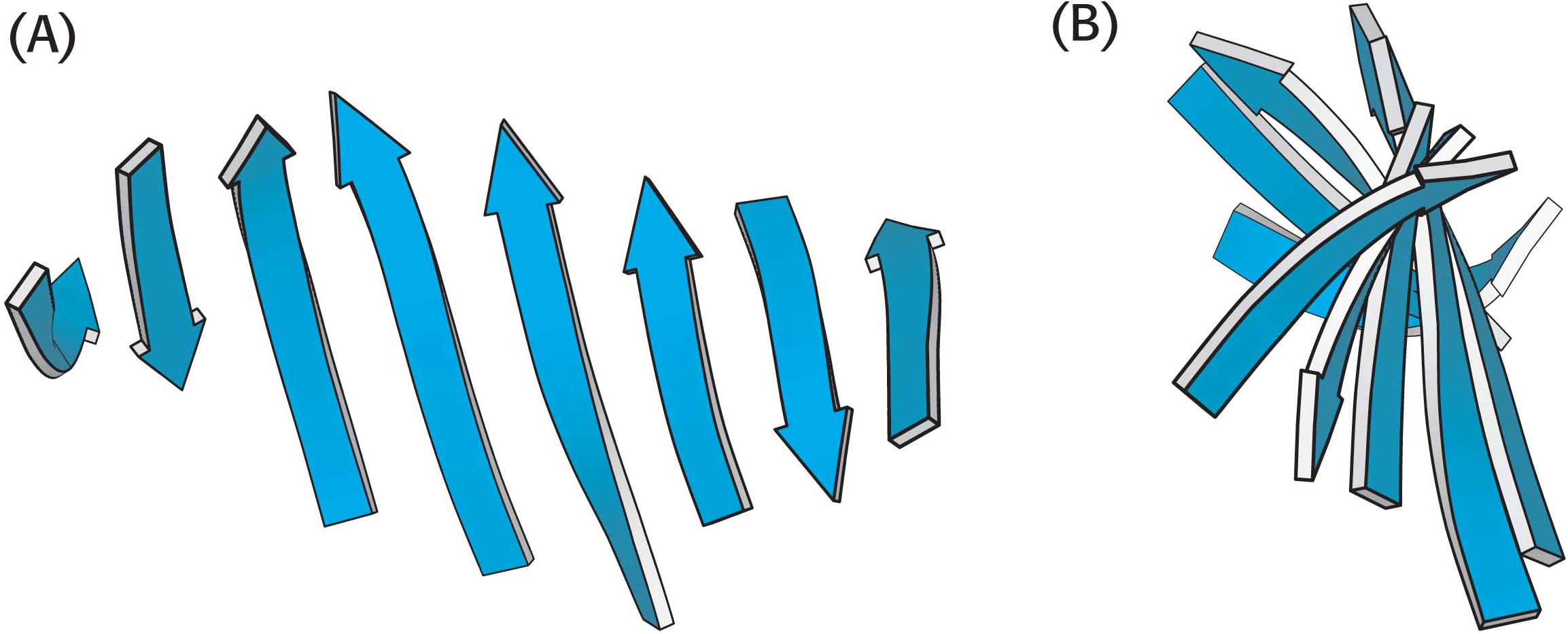
Figure 4.18: A twisted β sheet. (A) A schematic model. (B) The schematic view rotated by 90 degrees to illustrate the twist more clearly.
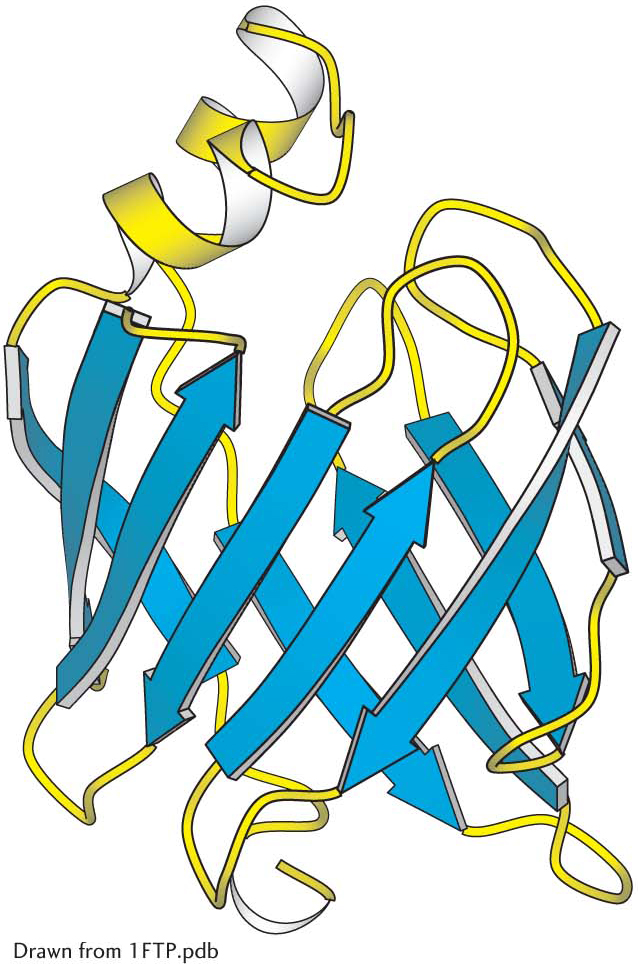
Figure 4.19:
A protein rich in β sheets. The structure of a fatty-acid-binding protein.
Fibrous Proteins Provide Structural Support for Cells and Tissues
Fibrous proteins form long fibers that serve a structural role. Although some of these proteins have regions of complex three-dimensional structure, for the most part, the three-dimensional structure of fibrous proteins is relatively simple, consisting of extensive stretches of secondary structure. Special types of helices are present in two common fibrous proteins, α-keratin and collagen. α-Keratin, which is the primary component of wool and hair, consists of two right-handed α helices intertwined to form a type of left-handed superhelix called a coiled coil. α-Keratin is a member of a superfamily of proteins referred to as coiled-coil proteins (Figure 4.21). In these proteins, two or more helices can entwine to form a very stable structure that can have a length of 1000 Å (100 nm) or more. Human beings have approximately 60 members of this family, including intermediate filaments and the muscle proteins myosin and tropomyosin. The two helices in α-Keratin are cross-linked by weak interactions such as van der Waals forces and ionic interactions. In addition, the two helices may be linked by disulfide bonds formed by neighboring cysteine residues.
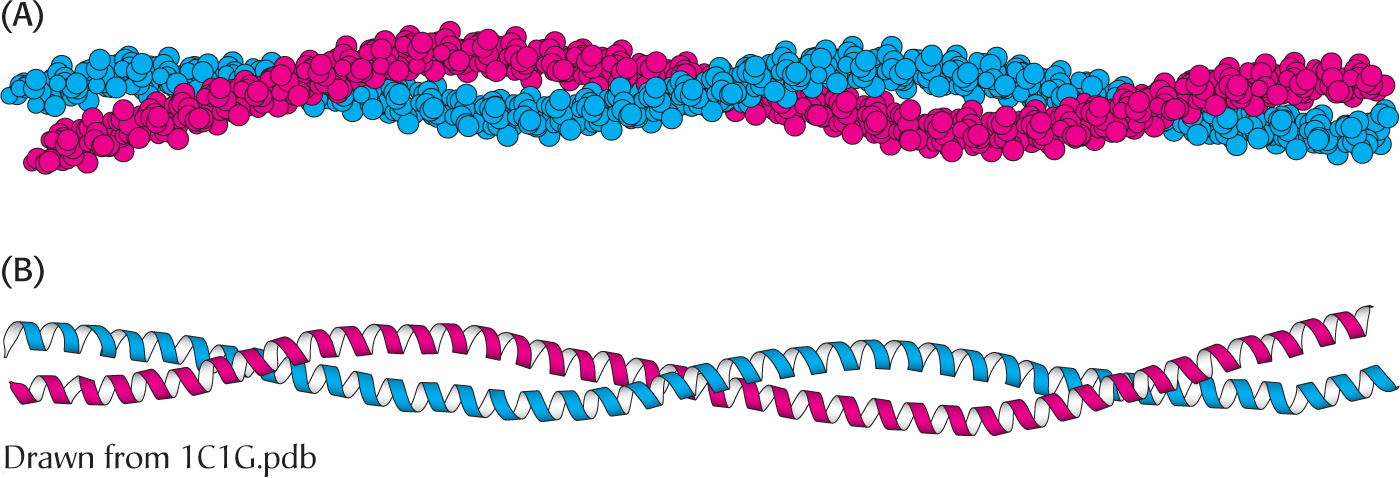
Figure 4.21: 
An α-helical coiled coil. (A) Space-filling model. (B) Ribbon diagram. The two helices wind around each other to form a superhelix. Such structures are found in many proteins, including keratin in hair, quills, claws, and horns.
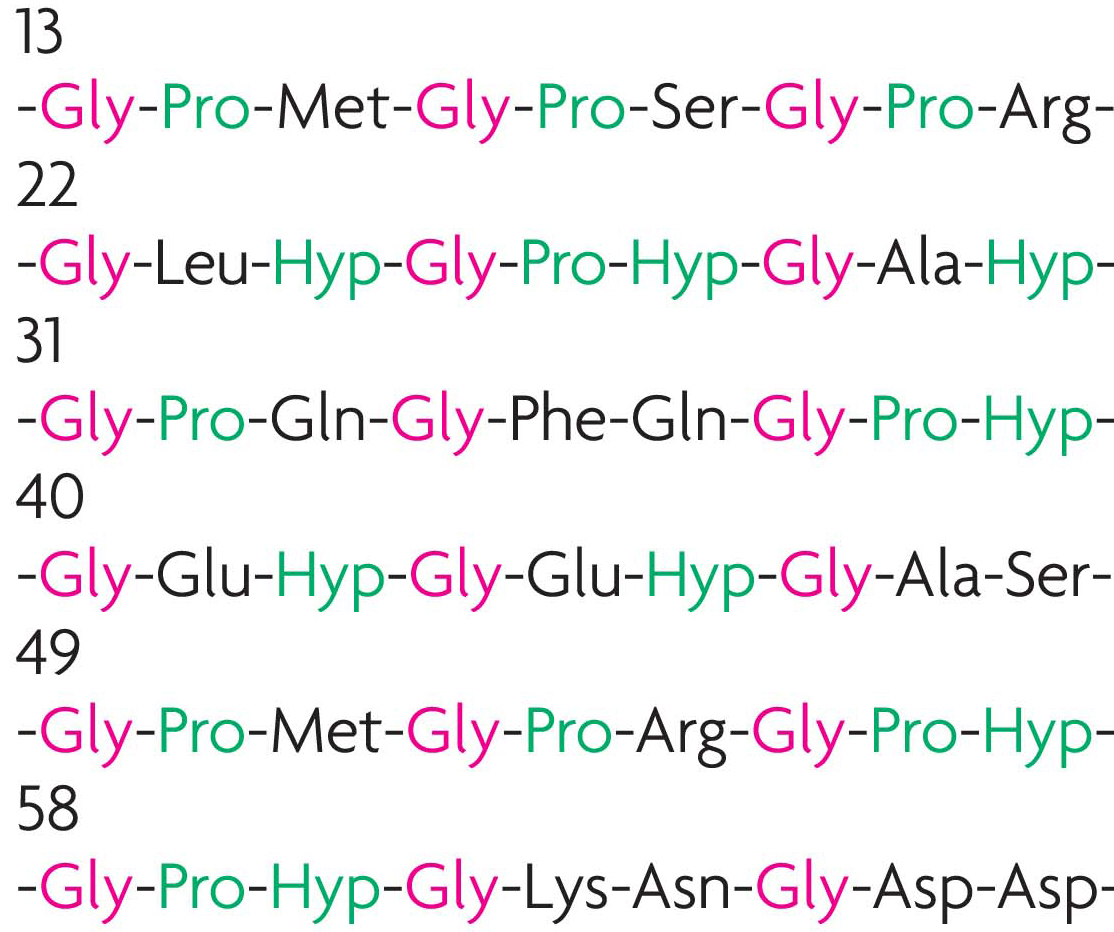
Figure 4.22: The amino acid sequence of a part of a collagen chain. Every third residue is glycine. Proline and hydroxyproline also are abundant.
A different type of helix is present in collagen, the most abundant mammalian protein. Collagen is the main fibrous component of skin, bone, tendon, cartilage, and teeth. It contains three helical polypeptide chains, each nearly 1000 residues long. Glycine appears at every third residue in the amino acid sequence, and the sequence glycine-proline-proline recurs frequently (Figure 4.22).
Hydrogen bonds within each peptide chain are absent in this type of helix. Instead, the helices are stabilized by steric repulsion of the pyrrolidine rings of the proline residues (Figure 4.23). The pyrrolidine rings keep out of each other’s way when the polypeptide chain assumes its helical form, which has about three residues per turn. Three strands wind around each other to form a superhelical cable that is stabilized by hydrogen bonds between strands. The hydrogen bonds form between the peptide NH groups of glycine residues and the CO groups of residues on the other chains. The inside of the triple-stranded helical cable is very crowded and explains why glycine has to be present at every third position on each strand: the only residue that can fit in an interior position is glycine (Figure 4.24A). The amino acid residue on either side of glycine is located on the outside of the cable, where there is room for the bulky rings of proline residues (Figure 4.24B).
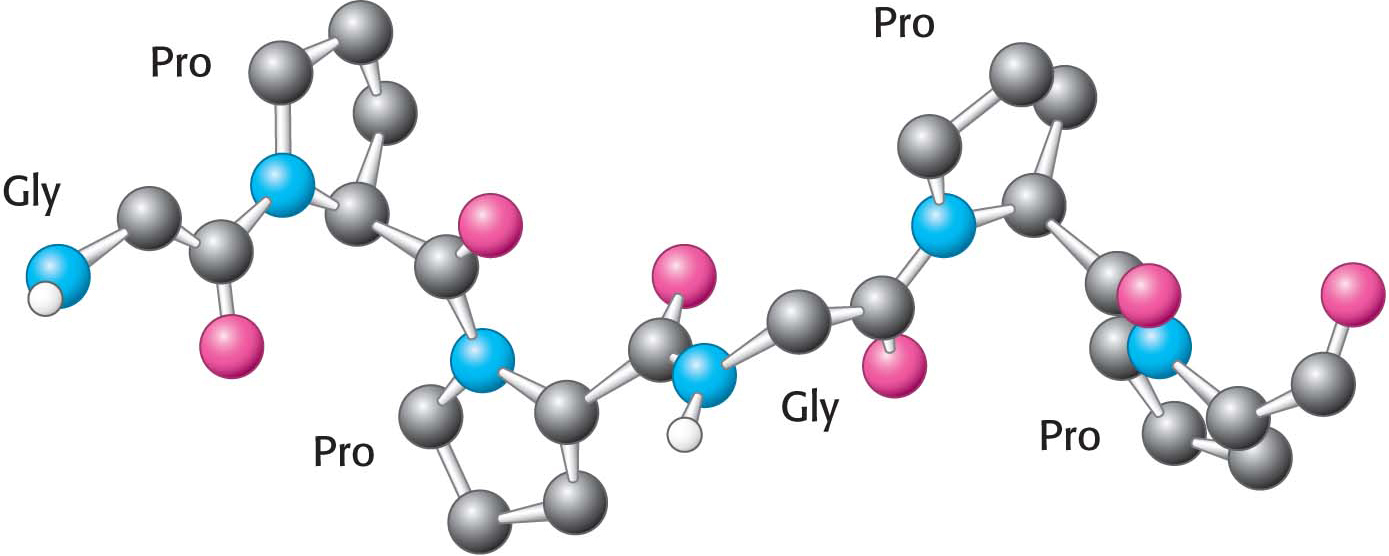
Figure 4.23: The conformation of a single strand of a collagen triple helix.
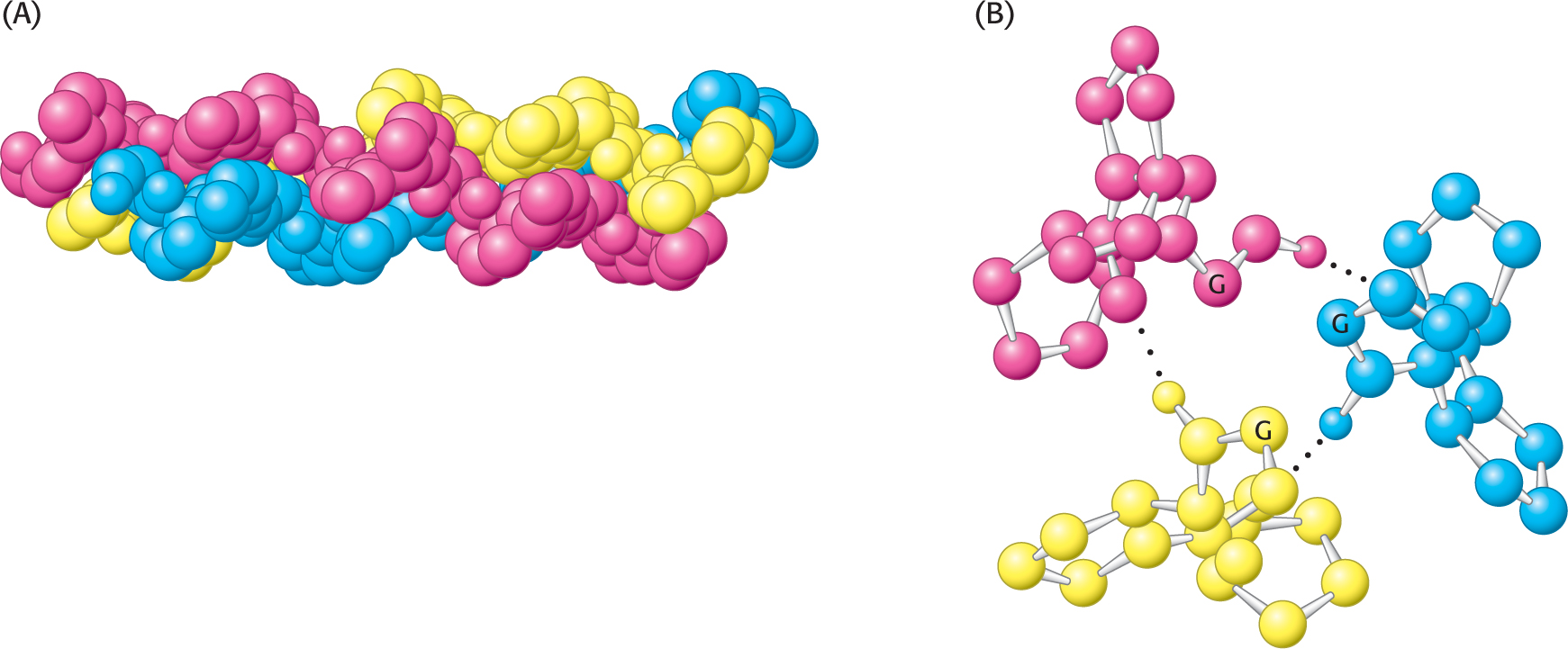
Figure 4.24: The structure of the protein collagen. (A) Space-filling model of collagen. Each strand is shown in a different color. (B) Cross section of a model of collagen. Each strand is hydrogen bonded to the other two strands. The atom of a glycine residue is identified by the letter G. Every third residue must be glycine because there is no space in the center of the helix. Notice that the pyrrolidine rings are on the outside.
NUTRITION FACTS: Vitamin C
Human beings are among the few mammals unable to synthesize vitamin C. Citrus products are the most common source of this vitamin. Vitamin C functions as a general antioxidant to reduce the presence of reactive oxygen species throughout the body. In addition, it serves as a specific antioxidant by maintaining metals, required by certain enzymes such as the enzyme that synthesizes hydroxyproline, in the reduced state.
!clinic! CLINICAL INSIGHT: Defects in Collagen Structure Result in Pathological Conditions
The importance of the positioning of glycine inside the triple helix is illustrated in the disorder osteogenesis imperfecta, also known as brittle bone disease. In this condition, which can vary from mild to very severe, other amino acids replace the internal glycine residue. This replacement leads to a delayed and improper folding of collagen, and the accumulation of defective collagen results in cell death. The most serious symptom is severe bone fragility. Defective collagen in the eyes causes the whites of the eyes to have a blue tint (blue sclera).
As we have seen, proline residues are important in creating the superhelical cable structure of collagen. Hydroxyproline is a modified version of proline, with a hydroxyl group replacing a hydrogen atom in the pyrrolidine ring. It is a common element of collagen, appearing in the glycine-proline-proline sequence as the second proline. Hydroxyproline is essential for stabilizing collagen, and its formation illustrates our dependence on vitamin C.
Vitamin C is required for the formation of stable collagen fibers because it assists in the formation of hydroxyproline from proline. Less stable collagen can result in scurvy. The symptoms of scurvy include skin lesions and blood-vessel fragility. Most notable are bleeding gums, the loss of teeth, and periodontal infections. Gums are especially sensitive to a lack of vitamin C because the collagen in gums turns over rapidly. Vitamin C is required for the continued activity of prolyl hydroxylase, which synthesizes hydroxyproline.
This reaction requires an Fe2+ ion to activate O2. This iron ion, embedded in prolyl hydroxylase, is susceptible to oxidation, which inactivates the enzyme. How is the enzyme made active again? Ascorbate (vitamin C) comes to the rescue by reducing the Fe3+ of the inactivated enzyme. Thus, ascorbate serves here as a specific antioxidant.