16.1 Glycolysis Is an Energy-Conversion Pathway
✓ 1 Describe how ATP is generated in glycolysis.
We now begin our consideration of the glycolytic pathway. This pathway is common to virtually all cells, both prokaryotic and eukaryotic. In eukaryotic cells, glycolysis takes place in the cytoplasm. Glucose is converted into two molecules of pyruvate with the concomitant generation of two molecules of ATP.
Glycolysis can be thought of as comprising two stages (Figure 16.1). Stage 1 is the trapping and preparation phase. No ATP is generated in this stage. Stage 1 begins with the conversion of glucose into fructose 1,6-bisphosphate, which consists of three steps: a phosphorylation, an isomerization, and a second phosphorylation reaction. The strategy of these initial steps in glycolysis is to trap the glucose in the cell and form a compound that can be readily cleaved into phosphorylated three-carbon units. Stage 1 is completed with the cleavage of the fructose 1,6-bisphosphate into two phosphorylated three-carbon fragments. These resulting three-carbon units are readily interconvertible. In stage 2, ATP is harvested when the three-carbon fragments are oxidized to pyruvate.
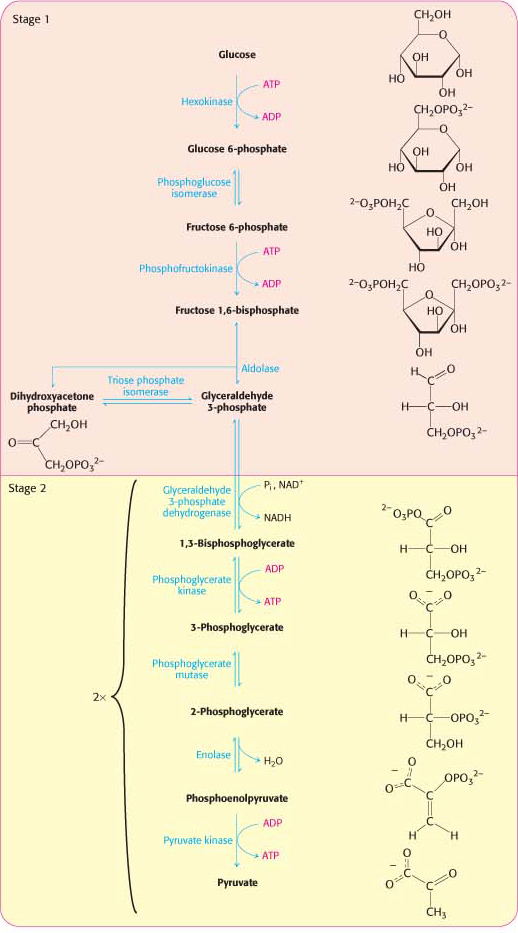
Figure 16.1: Stages of glycolysis. The glycolytic pathway can be divided into two stages: (1) glucose is trapped, destabilized, and cleaved into two interconvertible three-carbon molecules, generated by the cleavage of six-carbon fructose; and (2) the three-carbon units are oxidized to pyruvate, generating ATP.
Hexokinase Traps Glucose in the Cell and Begins Glycolysis
Glucose enters cells through specific transport proteins and has one principal fate inside the cell: it is phosphorylated by ATP to form glucose 6-phosphate. This step is notable for two reasons: (1) glucose 6-phosphate cannot pass through the membrane to the extracellular side, because it is not a substrate for the glucose transporters, and (2) the addition of the phosphoryl group facilitates the metabolism of glucose to phosphorylated three-carbon compounds with high phosphoryl-transfer potential. The transfer of the phosphoryl group from ATP to the hydroxyl group on carbon 6 of glucose is catalyzed by hexokinase:
Phosphoryl transfer is a fundamental reaction in biochemistry. Kinases are enzymes that catalyze the transfer of a phosphoryl group from ATP to an acceptor. Hexokinase, then, catalyzes the transfer of a phosphoryl group from ATP to a variety of six-carbon sugars (hexoses), such as glucose and mannose. Hexokinase, as well as all other kinases, requires Mg2+ (or another divalent metal ion such as Mn2+) for activity. The divalent metal ion forms a complex with ATP.
X-ray crystallographic studies of yeast hexokinase revealed that the binding of glucose induces a large conformational change in the enzyme. Hexokinase consists of two lobes, which move toward each other when glucose is bound (Figure 16.2). The cleft between the lobes closes, and the bound glucose becomes surrounded by protein, except for the carbon atom that will accept the phosphoryl group from ATP. The closing of the cleft in hexokinase is a striking example of the role of induced fit in enzyme action.
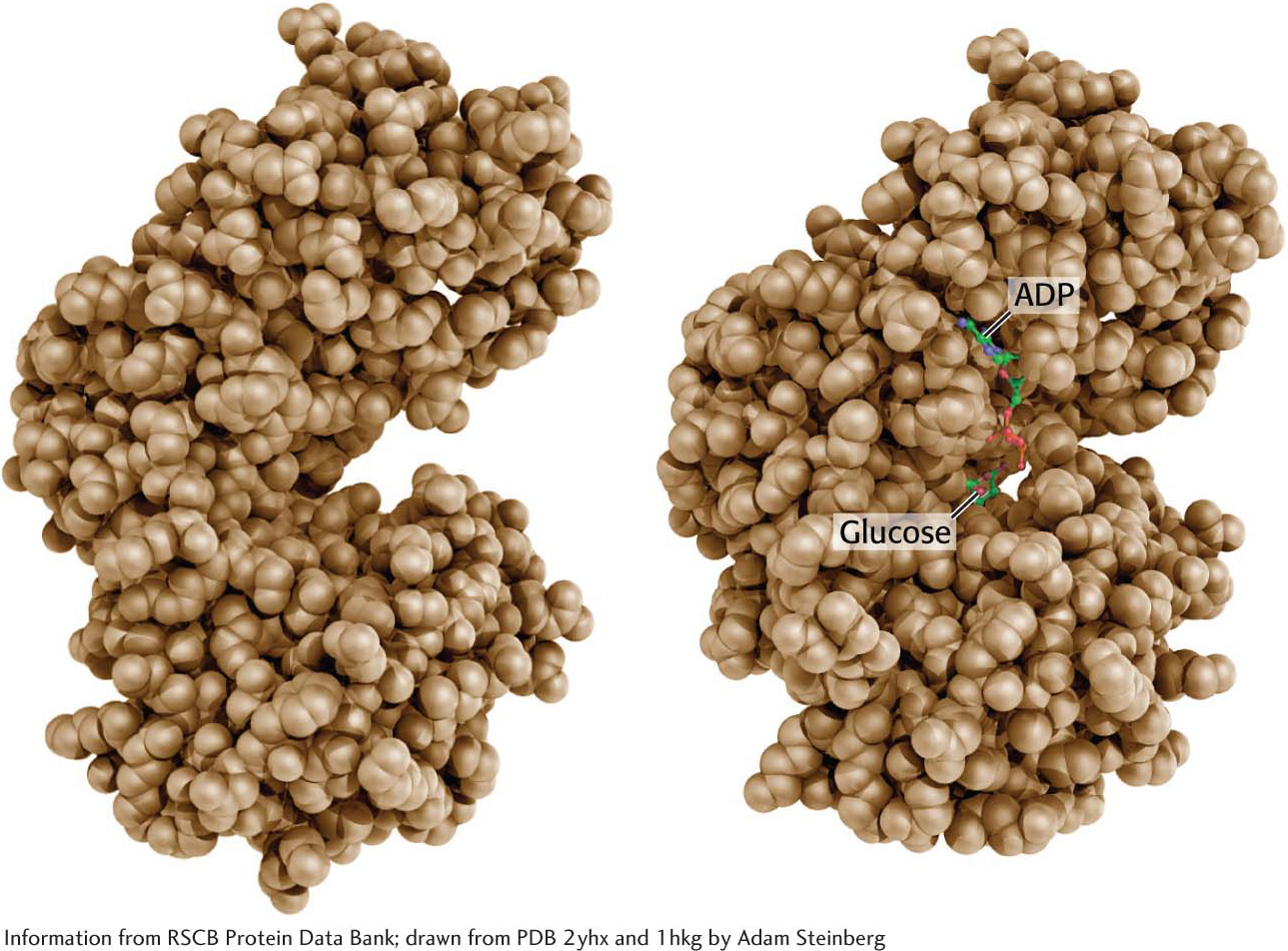
Figure 16.2: Induced fit in hexokinase. The two lobes of hexokinase are separated in the absence of glucose (left). The conformation of hexokinase changes markedly on binding glucose (right). Notice that two lobes of the enzyme come together, creating the necessary environment for catalysis.
Why are the structural changes in hexokinase of biochemical consequence? The environment around glucose becomes more nonpolar as water is extruded and the hydrophobic R groups of the protein surround the glucose molecule, which favors the donation of the terminal phosphoryl group of ATP. The removal of water from the active site enhances the specificity of the enzyme. If hexokinase were rigid, a molecule of H2O occupying, by chance, the binding site for the—CH2OH of glucose could attack the 7 phosphoryl group of ATP, forming ADP and Pi. In other words, a rigid kinase would likely also be an ATPase. Substrate-induced cleft closing is a general feature of kinases.
Fructose 1,6-bisphosphate Is Generated from Glucose 6-phosphate
The next step in glycolysis is the isomerization of glucose 6-phosphate to fructose 6-phosphate. Recall that the open-chain form of glucose has an aldehyde group at carbon 1, whereas the open-chain form of fructose has a keto group at carbon 2. Thus, the isomerization of glucose 6-phosphate to fructose 6-phosphate is a conversion of an aldose into a ketose. The reaction is catalyzed by phosphoglucose isomerase.
This isomerization is crucial because only three-carbon molecules are metabolized in the later stages of glycolysis. Glucose 6-phosphate is not readily cleaved into two three-carbon fragments, while fructose 6-phosphate is. A second phosphorylation reaction follows the isomerization step, trapping the sugar as the fructose isomer. Fructose 6-phosphate is phosphorylated by ATP to fructose 1,6-bisphosphate (F-l,6-BP):
DID YOU KNOW?
The prefix bis- in bisphosphate means that two separate monophosphoryl groups are present, whereas the prefix di- in diphosphate (as in adenosine diphosphate) means that two phosphoryl groups are present and are connected by an anhydride linkage.
This reaction, which is irreversible under cellular conditions, is catalyzed by phosphofructokinase (PFK), an allosteric enzyme that is the key regulatory enzyme for glycolysis.
!clinic! CLINICAL INSIGHT: The Six-Carbon Sugar Is Cleaved into Two Three-Carbon Fragments
The second stage of glycolysis begins with the cleavage of fructose 1,6-bisphosphate into two triose phosphates, glyceraldehyde 3-phosphate (GAP) and dihydroxyacetone phosphate (DHAP). The products of the remaining steps in glycolysis consist of three-carbon units rather than six-carbon units. This reaction, which is readily reversible, is catalyzed by aldolase:
Glyceraldehyde 3-phosphate is on the direct pathway of glycolysis, whereas dihydroxyacetone phosphate is not. These compounds are isomers that can be readily interconverted so as not to waste the useful three-carbon fragment that would be lost if dihydroxyacetone remained in its unusable form. The isomerization of these three-carbon phosphorylated sugars is catalyzed by triose phosphate isomerase (TPI, sometimes abbreviated TIM):
This reaction is rapid and reversible. At equilibrium, 96% of the triose phosphate is dihydroxyacetone phosphate. However, the reaction proceeds readily from dihydroxyacetone phosphate to glyceraldehyde 3-phosphate because the subsequent reactions of glycolysis remove this product. The importance of this reaction is demonstrated by the fact that TPI deficiency, a rare condition, is the only glycolytic enzymopathy that is lethal. This deficiency is characterized by severe hemolytic anemia and neurodegeneration.
The Oxidation of an Aldehyde Powers the Formation of a Compound Having High Phosphoryl-Transfer Potential
The preceding steps in glycolysis have transformed one molecule of glucose into two molecules of glyceraldehyde 3-phosphate, but no energy has yet been extracted. On the contrary, two molecules of ATP have been expended. We come now to the final stage of glycolysis, a series of steps that harvest some of the energy contained in glyceraldehyde 3-phosphate as ATP. The initial reaction in this sequence is the conversion of glyceraldehyde 3-phosphate into 1,3-bisphosphoglycerate (1,3-BPG), an oxidation–reduction reaction catalyzed by glyceraldehyde 3-phosphate dehydrogenase. Dehydrogenases are enzymes that catalyze oxidation-reduction reactions, often transferring a hydride ion from a donor molecule to NAD+ or transferring a hydride ion from NADH to an acceptor molecule:
1,3-Bisphosphoglycerate is an acyl phosphate, which is a mixed anhydride of phosphoric acid and a carboxylic acid. Such compounds have a high phosphoryl-transfer potential; one of its phosphoryl groups is transferred to ADP in the next step in glycolysis.
Let us consider this reaction in some detail because it illustrates the essence of energy transformation and metabolism itself: the energy of carbon oxidation is captured as high phosphoryl-transfer potential. The reaction catalyzed by glyceraldehyde 3-phosphate dehydrogenase can be viewed as the sum of two processes: the oxidation of the aldehyde (in this case, glyceraldehyde 3-phosphate) to a carboxylic acid by NAD+ and the joining of the carboxylic acid (3-phosphoglycerate) and orthophosphate to form the acyl-phosphate product, 1,3-bisphosphoglycerate:
The first reaction is thermodynamically quite favorable, with a standard free-energy change, ΔG°′, of approximately −50 kJ mol−1 (−12 kcal mol−1), whereas the second reaction is quite unfavorable, with a standard free-energy change of the same magnitude but the opposite sign. If these two reactions simply took place in succession, the second reaction would not take place at a biologically significant rate, because of its very large activation energy (Figure 16.3). These two processes must be coupled so that the favorable aldehyde oxidation can be used to drive the formation of the acyl phosphate. How are these reactions coupled? The key is an intermediate that is linked to the enzyme by a thioester after the aldehyde has been oxidized. This intermediate reacts with orthophosphate to form the high-energy compound 1,3-bisphosphoglycerate. The thioester is a free-energy intermediate between the aldehyde and the free carboxylic acid. The favorable oxidation and unfavorable phosphorylation reactions are coupled by the thioester intermediate, which preserves much of the free energy released in the oxidation reaction (Figure 16.3B).
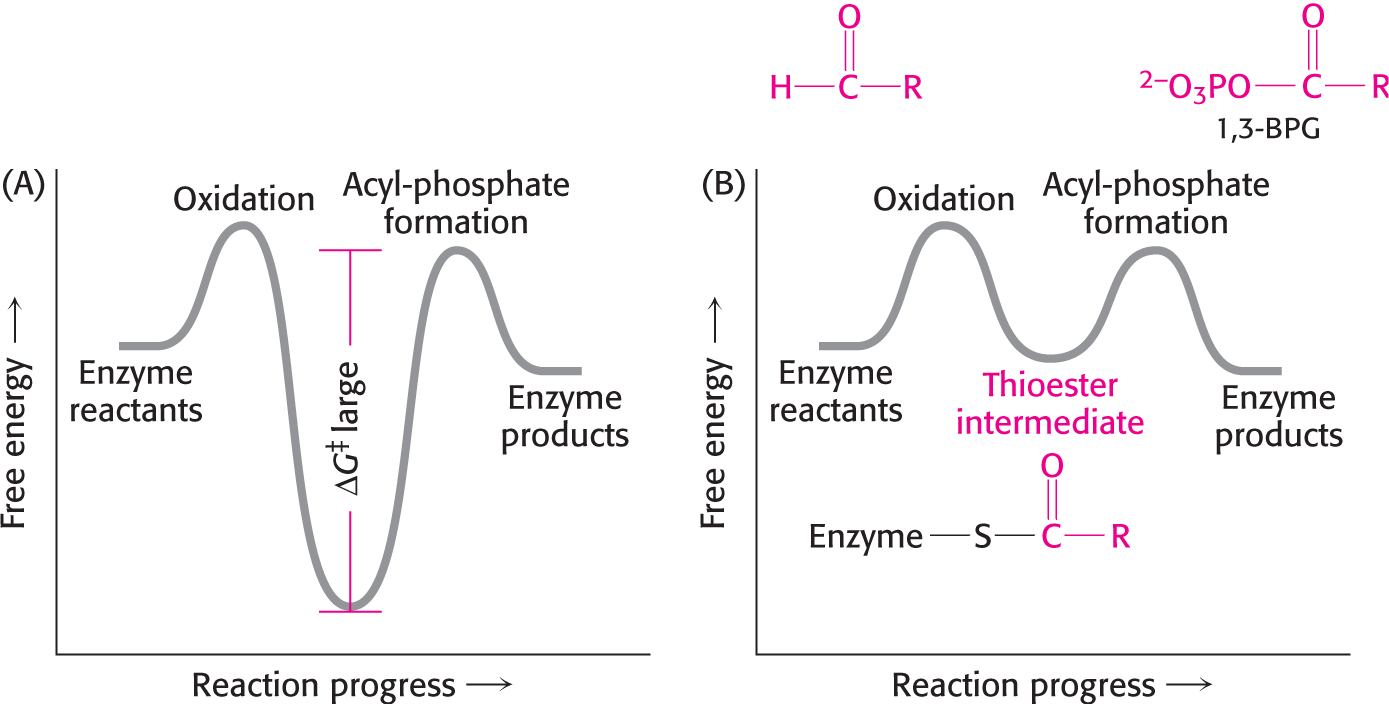
Figure 16.3: Free-energy profiles for glyceraldehyde oxidation followed by acyl-phosphate formation. (A) A hypothetical case with no coupling between the two processes. The second step must have a large activation barrier, making the reaction very slow. (B) The actual case with the two reactions coupled through a thioester intermediate. The thioester intermediate is more stable than the reactant, and, hence, its formation is spontaneous. However, the intermediate is less stable than the product, which forms spontaneously. Thus, the barrier separating oxidation from acyl-phosphate formation is eliminated.
ATP Is Formed by Phosphoryl Transfer from 1,3-Bisphosphoglycerate
1,3-Bisphosphoglycerate is an energy-rich molecule with a greater phosphoryl-transfer potential than that of ATP. Thus, 1,3-BPG can be used to power the synthesis of ATP from ADP and orthophosphate. Phosphoglycerate kinase catalyzes the transfer of the phosphoryl group from the acyl phosphate of 1,3-bisphosphoglycerate to ADP. ATP and 3-phosphoglycerate are the products:
The formation of ATP in this manner is referred to as substrate-level phosphorylation because the phosphate donor, 1,3-BPG, is a kinase substrate with high phosphoryl-transfer potential. We will contrast this manner of ATP formation with the formation of ATP from ionic gradients in Chapters 20 and 21.
We now start to see a return on our initial investment of two molecules of ATP in stage 1. Going backward in the pathway one step to glyceraldehyde 3-phosphate, we find the outcomes of the reactions catalyzed by glyceraldehyde 3-phosphate dehydrogenase and phosphoglycerate kinase to be as follows:
Glyceraldehyde 3-phosphate, an aldehyde, is oxidized to 3-phosphoglycerate, a carboxylic acid.
NAD+ is concomitantly reduced to NADH.
ATP is formed from Pi and ADP at the expense of carbon-oxidation energy.
In essence, the energy released in the oxidation of glyceraldehyde 3-phosphate to 3-phosphoglycerate is temporarily trapped as 1,3-bisphosphoglycerate. This energy powers the transfer of a phosphoryl group from 1,3-bisphosphoglycerate to ADP to yield ATP. Keep in mind that, because of the actions of aldolase and triose phosphate isomerase on fructose 1,6-bisphosphate at the end of stage 1, two molecules of glyceraldehyde 3-phosphate were formed and, hence, two molecules of ATP were generated. These ATP molecules make up for the two molecules of ATP consumed in the first stage of glycolysis.
Additional ATP Is Generated with the Formation of Pyruvate
In the remaining steps of glycolysis, 3-phosphoglycerate is converted into pyruvate, and a second molecule of ATP is formed from ADP:
The first reaction is a rearrangement. 3-Phosphoglycerate is converted into 2-phosphoglycerate by phosphoglycerate mutase, which shifts the position of the phosphoryl group. In general, a mutase is an enzyme that catalyzes the intramolecular shift of a chemical group, such as a phosphoryl group.
In the next reaction, the dehydration of 2-phosphoglycerate catalyzed by enolase introduces a double bond, creating an enol phosphate, an unstable class of molecule in relation to an alcohol such as 2-phosphoglycerate. Enolase catalyzes the formation of the enol phosphate phosphoenolpyruvate (PEP). This dehydration markedly elevates the transfer potential of the phosphoryl group.
Why does phosphoenolpyruvate have such a high phosphoryl-transfer potential? The phosphoryl group traps the molecule in its unstable enol form. When the phosphoryl group has been donated to ATP, the enol is able to undergo a conversion into the more stable ketone—namely, pyruvate. Hence, pyruvate is formed, and ATP is generated concomitantly. The irreversible transfer of a phosphoryl group from phosphoenolpyruvate to ADP is catalyzed by pyruvate kinase:
Because the two molecules of ATP used in forming fructose 1,6-bisphosphate were regenerated in the creation of two molecules of 3-phosphoglycerate, the two molecules of ATP generated from the two molecules of phosphoenolpyruvate are “profit.”
!quickquiz! QUICK QUIZ 1
The gross yield of ATP from the metabolism of glucose to two molecules of pyruvate is four molecules of ATP. However, the net yield is only two molecules of ATP. Why are the gross and net values different?
What is the energy source for the formation of phosphoenolpyruvate? The answer to this question becomes clear when we compare the structures of 2-phosphoglycerate and pyruvate. The formation of pyruvate from 2-phosphoglycerate is, in essence, an internal oxidation–reduction reaction; carbon 3 takes electrons from carbon 2 in the conversion of 2-phosphoglycerate into pyruvate. Compared with 2-phosphoglycerate, C-3 is more reduced in pyruvate, whereas C-2 is more oxidized. Once again, carbon oxidation powers the synthesis of a compound with high phosphoryl-transfer potential—phosphoenolpyruvate here and 1,3-bisphosphoglycerate earlier—which allows the synthesis of ATP.
Two ATP Molecules Are Formed in the Conversion of Glucose into Pyruvate
The net reaction in the transformation of glucose into pyruvate is
Thus, two molecules of ATP are generated in the conversion of glucose into two molecules of pyruvate. The reactions of glycolysis are summarized in Table 16.1 below.
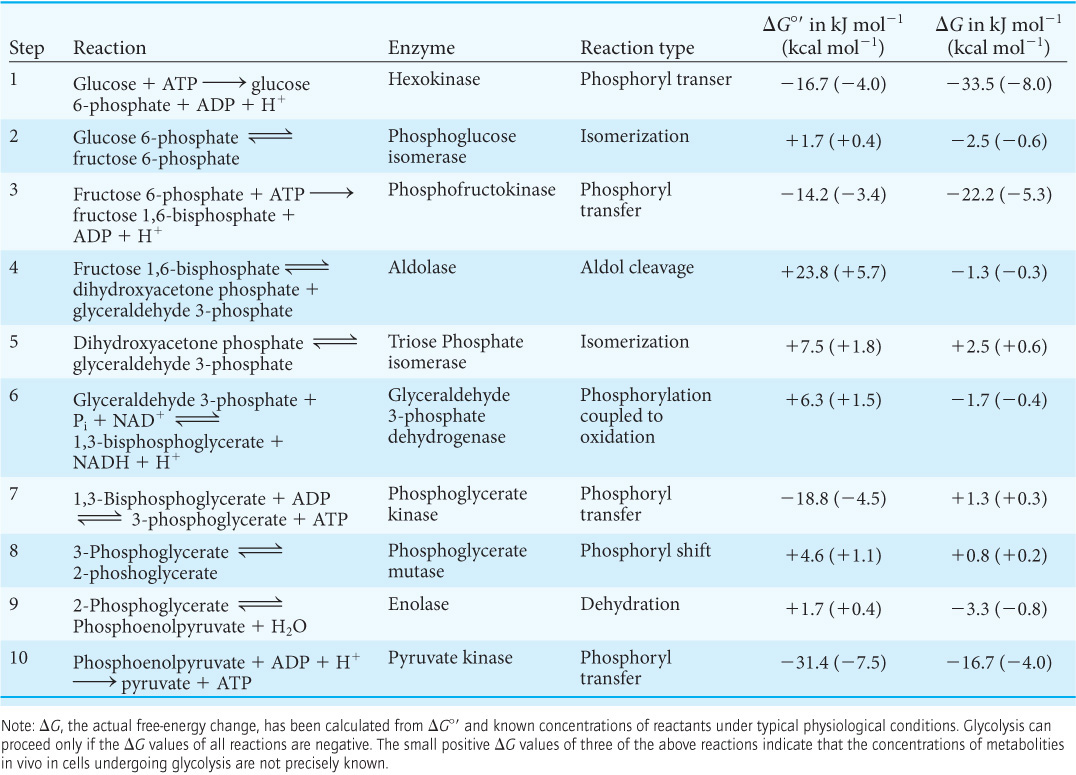
Table 16.1 Reactions of glycolysis
Note that the energy released in the anaerobic conversion of glucose into two molecules of pyruvate is about −90 kJ mol−1 (−22 kcal mol−1). We shall see in Chapters 20 and 21 that much more energy can be released from glucose in the presence of oxygen.